Previously thought impossible, researchers have found a way to create O type blood from other blood types. Since O is a universal donor, these other blood types can now be used as universal donors, potentially saving countless lives.
A team of biochemists from the University of British Columbia, lead by doctor Stephen Withers, have turned A positive and A negative type blood into the universal O type blood. These findings were published in June of 2019 in the journal “Nature Microbiology.”[1]
Before these findings, the 8 major blood types were not all compatible with each other. This meant that if a blood transfusion were needed for a patient, a specific type of blood maybe be needed, with no other type working.
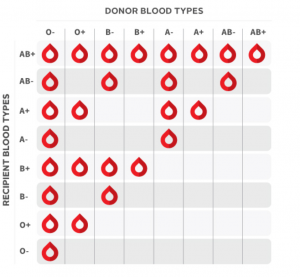
A chart of what blood types can donate or receive other blood types.[2]
This problem has existed since blood transfusions were first scientifically achieved, and scientists have been looking for a solution for just as long. It turns out the solution was hiding right under our noses; inside our stomachs, to be specific!
Inside the human gut are thousands of microscopic bacteria which we use to digest food and convert it into energy. As it turns out, these bacteria are very good at safely interacting with the human body in helpful ways. The researchers extracted these microorganisms through human feces and found they could be used in exactly the way they were hoping.
“Why would they be looking in our stomachs for this solution?” you might ask. The Withers group were on a hunt for a special kind of protein called an enzyme. Enzymes are produced by the body with a very specific task, and that task varies based on what the body wants in to do. In the below picture, notice how the enzyme matches perfectly the substrate. This is because enzymes are made for specific purposes, and only match specific molecules in the body. Since our gut has the ability to process blood and turn it into energy, Withers and his team decided to see if these enzymes could be harnessed for their research. As it turns out, they were completely correct.
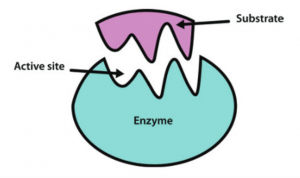
An enzyme interacting with a specific molecule (known as the substrate) in the body.[3]
In the United States and Canada alone, 4.5 million patients need blood transfusions every year.[4] This high demand means that often times, the supply cannot meet the demand. With this new discovery, more blood types are compatible, which means more people can be helped.
While the process has been completed in the lab, it has yet to be scaled up to convert massive amounts of blood at a time. This may take some time to accomplish. However, it is impossible to quantify exactly how many people this new method will help, or even how many lives it will save. One thing is for certain, is that the world of blood donations will forever feel the impact of these findings.
References:
- Rahfeld, P., Sim, L., Moon, H., Constantinescu, I., Morgan-Lang, C., Steven, J. H., Kizhakkedathu, J. N., Withers, S. G.; An enzymatic pathway in the human gut microbiome that converts A to universal O type blood. Nature Microbiology. 2019, 1475-1485.
- Canadian Blood Services. Do You Know Your Blood Type? https://blood.ca/en/blood/donating-blood/whats-my-blood-type (accessed March 21, 2020)
- Western Oregon University: Chemistry. CH450 and CH451: Biochemistry – Defining Life at the Molecular Level. Chapter 6: Enzyme Principles and Biotechnological Applications. https://wou.edu/chemistry/courses/online-chemistry-textbooks/ch450-and-ch451-biochemistry-defining-life-at-the-molecular-level/chapter-7-enzyme-kinetics/ (accessed Mar 21, 2020)
- Community Blood Bank of Northwest Pennsylvania and Western New York. 56 facts about blood. https://fourhearts.org/facts/ (accessed March 22, 2020)