A journal article published by Nature in June 2019, studied a novel method in converting type A blood to the universal type O blood using bacteria found in poop! The Withers’ research group at the University of British Columbia used the DNA of bacteria found in the faecal samples to develop this efficient method.
HOW ARE BLOOD TYPES CLASSIFIED?
There are four main blood types: A, B, AB, O.
Blood type is determined by the type of antigens and antibodies present in the blood. Antibodies are part of your body’s defense system, if they sense germs (or any other foreign substance) they alert your immune system which stimulates a biological response to destroy said germs.
Antigens are proteins that are directly attached to red blood cells that are used to identify blood as A, B, AB or O. Antigens are also attached to foreign substances, and antibodies use them as indicators that a substance does not belong in the body. Figure 1 shows how antibodies interact with antigens.
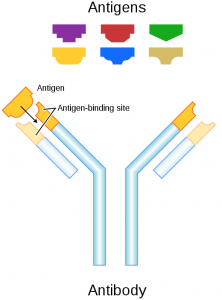
Figure 1: Image of antibody interacting with an antigen. Obtained from Wikipedia commons.
For example, if you have type A blood then your red blood cells will have A antigens. However, your blood will have type B antibodies. This means that the immune system will attack any substance with B antibodies – including type B blood. This explains why individuals who get blood transfusions can only accept their same blood type, unless it is the universal type O.
Universal type O blood doesn’t have any antigens so anyone can accept O blood as their antibodies will not attack the red blood cells. Figure 2 shows the different blood groups and how they are classified.
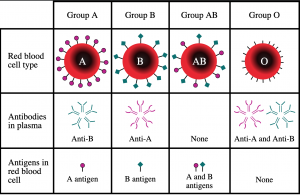
Figure 2: Image of different blood types depicting what antigens and antibodies they contain. Obtained from Wikipedia commons.
RESULTS OF THE STUDY
Figure 3 shows an in depth depiction of the difference in antigens between the blood groups.
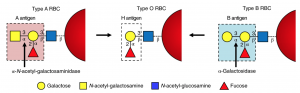
Figure 3: In depth image depicting the different constituents of antigens on type A, O and B blood. Obtained from journal article “An enzymatic pathway in the human gut microbiome that converts A to universal O type blood”
The researchers of this study, synthesized enzymes from the bacterial DNA found in poop. Enzymes are a type of protein that aid in the reactivity of a reaction, essentially meaning they help a reaction occur faster by breaking down substances. Figure 4 demonstrates how enzymes break down substances known as substrates.
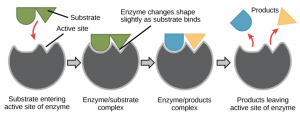
Figure 4: Image depicting how enzymes break down substances into two products. Obtained from Wikipedia commons.
In this case, the researches synthesized an enzyme that break down the bond labelled ‘3-α’ in type A and B blood cells (Figure 3). Once this bond is broken down, you are left with type O blood. The enzyme that Dr. Stephen Withers group identified, breaks down this bond 30 times faster than the previous candidate.
WHAT ARE THE IMPLICATIONS?
In January 2020, the American Red Cross announced that it has a ‘critical’ shortage of type O blood.
With this new method, hospitals and relief organizations, such as the American Red Cross, can convert type A and B blood to the universal O. It is vital that hospitals and relief organizations have a constant supply of O blood in case they need to do blood transfusions without knowing the blood type of the patient. It could also completely eliminate the need for blood type compatibility, if the research leaves the lab.
This enzyme is 30 times faster and, thus, more efficient than the previous candidate. This means that less of this enzyme is required to convert the same amount of blood, drastically decreasing the cost of production.
Overall, not only is this new method interesting to the science community but is is also incredibly important to the healthcare system.
-Chantell Jansz