My reflections on this course begin with recollecting my original motivations and professional context. I took this course to learn more about integrating technology into my math courses and better support student learning. I like learning new ideas and techniques that can improve my practice. My posts represent me, an experienced math teacher, and my work with adults in an essential skills program as they strive to further their work opportunities and build a better future. My learners and I are practical, and my posts reflect my realist perspective accompanied by a healthy dash of skepticism. My ideas blend professional experiences, literature, and my desire to build on my current best practices and those new to me.
I have organized my e-folio analysis according to three recurring themes in my posts:
- Theme 1: The Professional Teacher’s Knowledge, Skills, and Attributes
- Theme 2: Technological Tools and Their Affordances
- Theme 3: Opportunities and Challenges
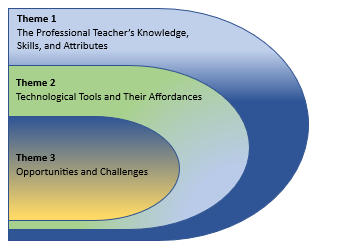
These themes are interwoven throughout my posts and are sometimes hard to isolate from each other. Rather than each standing discreetly, it becomes more of a shift between lenses and what emerges in the foreground and what shifts to the background.
I have been a teacher for 36 years and never felt like I had it all mastered! The truth is that teaching is a continual process of learning, applying, and adapting to deliver a learning experience that is effective, lasting, and enjoyable for learners while empowering them to lead fulfilling lives. My posts reside in the world of technology, pedagogy, and content knowledge (TPACK) and reinforce the importance of teachers developing this area to build a broad set of skills. The following excerpt is my response to a query about how I would characterize my teaching strategies:
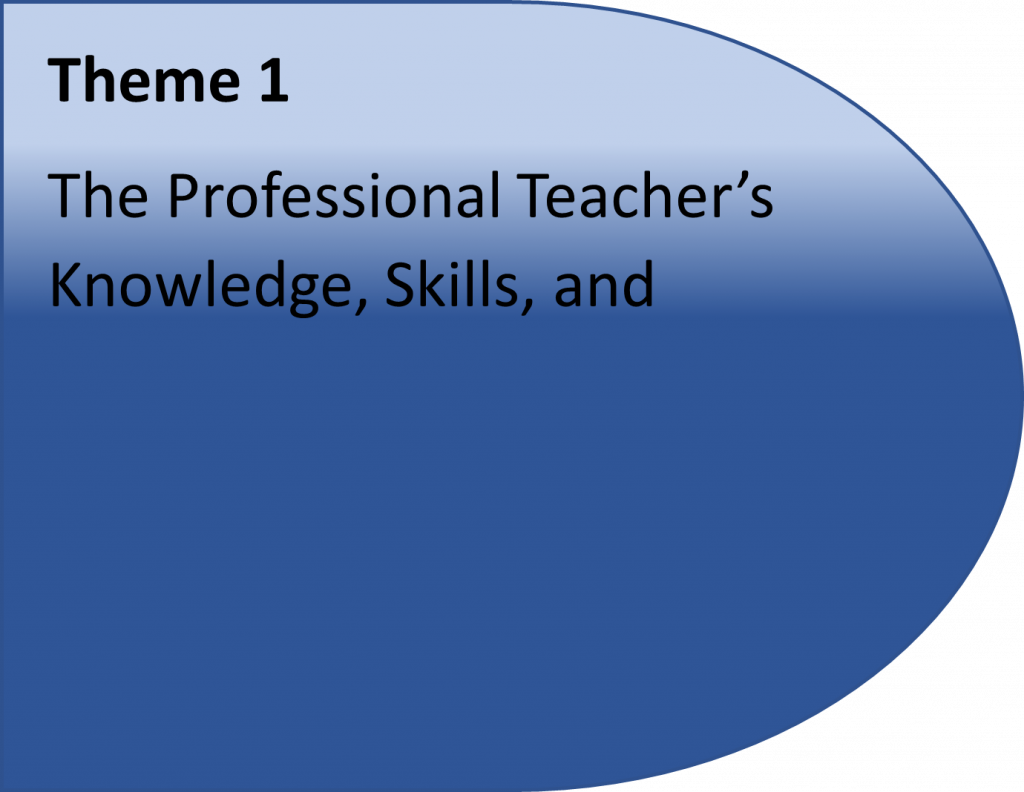
I have been a teacher for 36 years and never felt like I had it all mastered! The truth is that teaching is a continual process of learning, applying, and adapting to deliver a learning experience that is effective, lasting, and enjoyable for learners while empowering them to lead fulfilling lives.
My posts reside in the world of technology, pedagogy, and content knowledge (TPACK) and reinforce the importance of teachers developing this area to build a broad set of skills. The following excerpt is my response to a query about how I would characterize my teaching strategies:
I believe all good teaching designs reflect a combination of different strategies based on different learning theories, and that selecting what approach to use when, depending on the objectives, context, and constraints, is what the TPACK framework encapsulates. In lay terms, we can’t always hammer square pegs into round holes. Ertmer & Newby (2013) describe the instructional design challenge more eloquently as a quest to find “the optimal means for achieving desired outcomes” and identify a critical factor as being the degree of cognitive processing the task requires. Snelbecker (1983, as cited in Ertmer and Newby, 2013) encourages designers to use different tools, emphasizing that they cannot adopt the “luxury of restricting themselves to only one theoretical position… [They] are urged to examine each of the basic science theories which have been developed by psychologists in the study of learning and to select those principles and conceptions which seem to be of value for one’s particular educational situation” (p. 8).
(Thomas, 2023a, TPACK Discussion)
I am always looking for alternate strategies to consider and apply if they serve the purpose better. My posts reflect my continued investigation and exploration of how technology integrates into my practice and context. Figure 1 effectively summarizes the complexity of factors involved and my position that “as teachers we need to be critical about why we adopt new technology, and not [readily] succumb to the ‘new is better’ philosophy that the developers promote as part of their market capture” (Thomas, 2023a, Viewing the Cases Discussion).
Figure 1.
The emerging four factors affecting primary teachers’ technology integration (Spiteri & Rundgren, 2020)
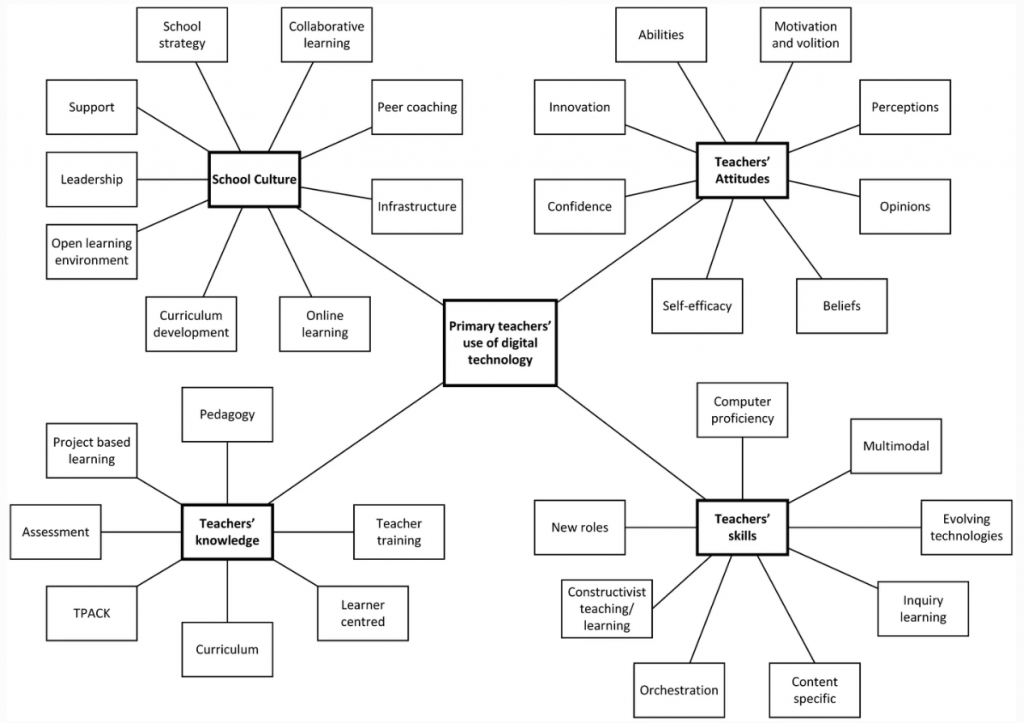
The TPACK framework to structure my thinking has also emphasized my belief and position that a significant amount of teaching is in designing activities. Technology integration shifts the role of the teacher to the sidelines, but it does not diminish the role of the teacher. Learning in the classroom still demands teacher presence to direct, support, and extend student learning. The teacher continues to build a student-to-teacher connection that is vital to effective learning. Although this comment is contextualized in Khan’s (2012) discussion of the T-GEM approach, I suggest it applies to technology tools in general,
[T]he web applications, on their own, may not be effective at supporting learning since they may lack guided steps, probing questions, or support problem solving. Thus, with T-GEM the teacher role is clearly tasked with supporting the learning at each phase of the T-GEM cycle.
(Thomas, 2023a, T-GEM Discussion)
Even when tools might not align with my teaching objectives and strategies, such as the ARCGIS toolkit, I identify the TPACK elements that do, such as its blend of cognitive and constructivist practices and “the 3 design steps that the LfU model uses (Edelson, 2001) in [similarity to] my own practice, specifically, establishing motivation, scaffolding new knowledge through guided exploration, and establishing a relevant and meaningful context” (Thomas, 2023a, LfU Discussion).
My posts frequently reference learning environments’ social, emotional, and cognitive components. Awareness of these components allows for a balanced approach to learning. Although the different features do not receive equal attention, various parts are identified and considered for different objectives and contexts. These learning components are also interrelated, and development in one area can impact development in others. As I examine my journey, it is interesting to note that my first and last posts expounded on emotional elements. I describe my recollection of the logarithmic ruler, “I was curious and mystified about how the manual contraption could be solving their calculations” (Thomas, 2023a, Auto E-ography Discussion). Later, I share the work of a former colleague as she reveals, “As I continue to learn and grow in this exciting space, I get ever more convinced of the power of immersive [VR] technology to enhance people’s sense of awe, wonder, and empathy for this planet and its inhabitants” (Edwards, 2022). Both reflections emphasize the importance of emotion in driving learning and development in the cognitive and social spheres.
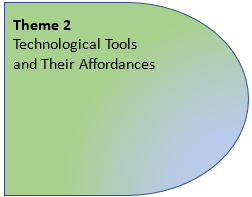
Educational tools have various affordances that can benefit my students. Recognizing the affordances incentivizes me to integrate these tools into meaningful learning activities. Here I summarize the affordances I have identified throughout the course in my posts.
Table 1
Technology-Enhanced Learning Environments (TELEs) Affordances
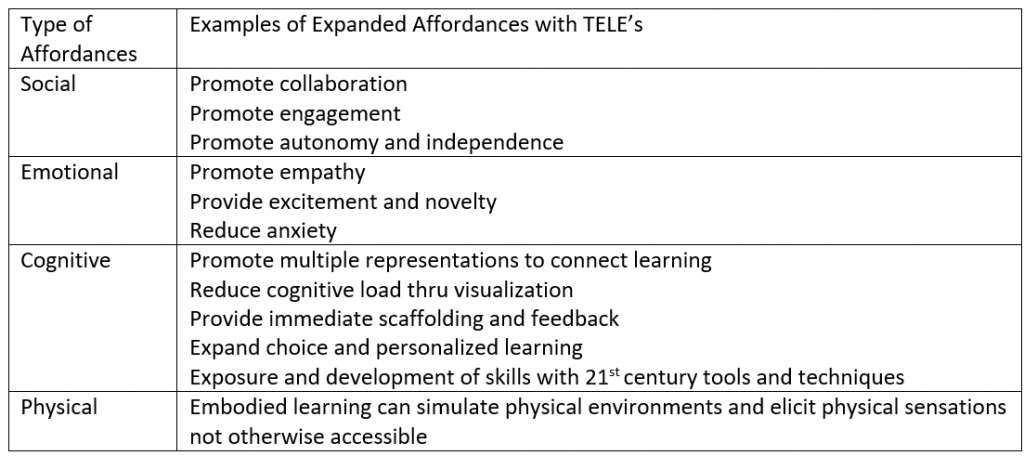
Throughout this course, I focused on educational technology tools useful in my immediate math teaching context. Even though educators, researchers, or learners endorse the tools, each teacher must evaluate them in the context of their unique learning goals. While technological devices can be amazing, they are always applicable, and I have created my own hot or not list. One of the most important features for making my list is that the tools are easy to learn and can integrate into my current classroom activities with minimal adjustment. My hot list for visualizations includes Desmos, GeoGebra, and Mathigon. These tools effectively illustrate math concepts and support problem-solving and communication. The following excerpts represent some thought around how I can make use of them.
I also like that Desmos can be used to create a polished graph so easily, …, shared with the class, and the resulting equation discussed – and so easily. … I would provide a computer lab setting for this activity, and introduce learners to the Desmos activity in a paired learning/collaborative learning space. I would also have physical handouts …which would act as scaffolds.
(Thomas, 2023a, T-GEM Discussion)
GeoGebra’s activities afford active, student-centered, discovery-based experiences that can be completed individually or in groups. … Mathigon can be used by the student for active and discovery-based learning, or it can be used by the teacher to help illustrate concepts and procedures, or both.
(Thomas, 2023a, Padlet Resource Sharing Discussion)
A method I use, reinforced throughout the course, involves using video presentations for learners who need more time, prefer greater independence, and benefit from multiple modalities or choice of delivery. Roblyer & Doering’s (2012) statement reiterates this, “technology can support multiple paths to studying the same material (p.42).”
(Thomas, 2023a, Anchored Instruction Discussion).
Additionally, I have identified engagement tools such as Quizlet, Quizizz, Kahoot, and Poll Everywhere that are useful for formative assessment, learning reinforcement, active learning, and team-building. I agree with my interviewee that “… for the most part students like it. They like the way that it breaks up the class, and it’s something different; it can be fun. But you’ve got to be careful not to overuse it” (Teacher T, 2023, as cited in Thomas, 2023b).
There are some tools that I don’t anticipate using, such as TinkerCAD or ArcGIS, which for my learners, would require too much time to learn and would not likely be continued beyond the course. Similarly, AR and VR tools look exciting, but their specialized nature and high cost make them a lower priority. I am committed to providing quality learning experiences for my students through the effective identification and integration of educational technology tools, which offer multiple benefits that would otherwise not be achievable
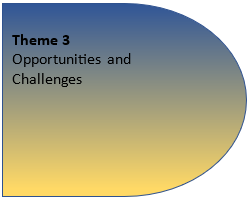
The final theme in my course journey is identifying the opportunities and challenges that implementing TELEs presents.
My Unpacking Assumptions post outlined a lengthy list of what I expect of good technology tools; this course has demonstrated the ability of technology to deliver. TELEs are capable of providing student-centered, flexible, and engaging activities. The internet connects learners with each other and a broader community while expanding available real-life contexts, thus enhancing meaning and transference to the material covered. Integrating technology improves the efficiency and effectiveness of various tasks and reflects practices that apply in 21st-century workplace settings. This extensive list of benefits might compel one to make a significant shift in practice, but I routinely ground myself in realistic undertakings, reminding myself and others that,
You don’t have to go all in with TPACK to gain something from it. Whether you apply it to every lesson or revisit it from time to time, this framework can help you think more strategically about how you’re using technology in the classroom.
(PowerSchool, 2023)
In my teacher interview reflection, I note this shift in my perspective, “At the onset, based on the complexity I envisioned [in integrating technology], teacher time commitment to was a significant issue in my mind. Now I recognize that integrating technology effectively does not require dramatic and instantaneous change” (Thomas, 2023b). I have evolved in my thinking and note, “Goals need to be SMART; progress can be small and incremental and still make a difference to one’s practice and students’ learning” (Thomas, 2023a, Knowledge Mobilization Discussion)
I think that there is more to think about than the technology when adopting new ed tech into practice, such as taking the time to identify the purpose and best manner to integrate. Having said that, sometimes you just need to dive in and give it a try so you can make those judgments.
(Thomas, 2023a, TPACK Discussion)
Alternatively, I am aware that these opportunities are not without potential barriers and complications, and understanding and anticipating them allows one to strategize to either mitigate or accept them. Teachers and students will need training to work with new technology, which will require time and energy. Student partnerships and teacher collaborations can provide valuable support to muddle through various challenges.
Assumptions around anticipated challenges, such as low digital literacy and limited device access, can be minimized by providing technological support and facilitating access to the necessary technology. Possible learner reluctance to try new instructional and technical strategies can be moderated best in a face-to-face environment first and can improve learners’ overall experience and adoption. Activity designs that integrate TPACK’s essential elements intentionally, appropriately, effectively, and flexibly will be well-positioned to develop Roblyer & Doering’s (2012) view that technology is valuable in solving problems and developing 21st-century skills and attitudes.
(Thomas, 2023a, Anchored Instruction Discussion)
This one was one of my biggest frustrations at the onset of the course, but I have recognized that I need to be realistic in setting and reaching goals and need to adopt a more generous timeline. The solution, derived from my teacher interview, is to recognize that good things take time. “Similar to GEM, teaching is a practice that improves with repeated iterations” (Thomas, 2023a, Synthesis Discussion).
My frustrations and expectations around student adoption of technology have also softened. Technology integration may be exceptionally challenging for students with fewer skills and less access. Thus, it is vital to provide encouragement, support, and alternatives to prevent technology from becoming a barrier.
DiGiusto (n.d., as cited in Vilchis, 2022) provides three guidelines for incorporating technology:
(Thomas, 2023a, Unpacking Assumptions Discussion)
- The technology should be optional, allowing students to appreciate the benefits and adopt it by choice.
- It must be inclusive in a social setting, promoting connections and generating resources that increase the learning quality without alienating the students.
- It should enrich student-teacher interactions.
This analytical reflection captures my interests, intentions, and strategies for further exploration as I transition from here to my teaching context. Technology integration is an aspect I will continue exploring, adapting, and improving with my teaching practice, using the TPACK framework, my expanded awareness of technological tools, and my desire to continuously evolve my practice to serve students better.
References
Edelson, D. C. (2001). Learning-for-use: A framework for the design of technology-supported inquiry activities. Journal of Research in Science Teaching, 38(3), 355-385.
Edwards, L. (2022, December 18). An orca carousel feeding scene. Found in the Fjords https://foundinthefjords.org/
Ertmer, P. A., & Newby, T. J. (2013). Behaviorism, cognitivism, constructivism: Comparing critical features from an instructional design perspective. Performance Improvement Quarterly, 26(2), 43-71.
Khan, S. (2012). A Hidden GEM: A pedagogical approach to using technology to teach global warming. Source. The Science Teacher, 79(8), 59-62. https://www.jstor.org/stable/43557744
PowerSchool. (2022, April 20). The TPACK Framework Explained (With Classroom Examples). PowerSchool. Retrieved February 8, 2023 from https://www.powerschool.com/blog/the-tpack-framework-explained-with-classroom-examples/#:~:text=TPACK%20is%20a%20technology%20integration,content%20knowledge%20(a.k.a.%20TPACK)
Roblyer, M. D., & Doering, A. H. (2012). Integrating Educational Technology into Teaching. (6th Edition ed.) Allyn & Bacon.
Snelbecker, G. E. (1989). Contrasting and complementary approaches to instructional design. In C. M. Reigeluth (Ed.), Instructional theories in action (pp, 321-337). Hillsdale, NJ: Lawrence Erlbaum Associates.
Spiteri, M., & Chang, Rundgren, S. (2020). Literature review on the factors affecting primary teachers’ use of digital technology. Technology, Knowledge and Learning, 25(1), 115-128. https://doi.org/10.1007/s10758-018-9376-x
Thomas, B. (2023a, Various Dates). Re: Various posts [Discussion post]. UBC ETEC533-65A Technology in the Mathematics Science Classroom 2022W2 EdXEdge. https://learning.edge.edx.org/course/course-v1:UBC+ETEC533-65A+2022W2/home
Thomas B. (2023b, February 2). Implementing Technology in the Classroom: A Teacher’s Perspective. ETEC 533 Brenda Thomas. https://blogs.ubc.ca/etec533brendathomas/
Vilchis, N. (2022). The best way to adopt EdTech is to do it with purpose. Edu News. https://observatory.tec.mx/edu-news/educational-technology-with-purpose/