To combat climate change and minimize greenhouse gas (GHG) emissions, governments at different levels all around the world have invested in research and new technology to reduce the emissions within their jurisdiction. Since most the GHGs are emitted from dense cities, a lot of attention has been given to reducing emissions from infrastructure within cities such as transportation, buildings, waste treatment and other infrastructures.
Making the change to low carbon infrastructure, an infrastructure that emits less greenhouse gasses than it sequesters or offsets, is deemed difficult and expensive within cities that are already fully developed and populated. With that logic, the idea of building a city from ground up that emits little to zero GHGs seems very appealing. Perhaps in line with Elon Musk’s vision to abandon the civilization that is already created and start a brand new one on Mars!
That is exactly what the government of the United Arab Emirates (UAE) planned to do in 2006. The city of Masdar, a zero-carbon city that is fuelled completely by renewable energy and will house more than 40,000 residents and accommodate for another 50,000 commuters who will work in the city by 2016. The idea and the design of the city was truly revolutionary. In this post, I will discuss a few of the major features of the city and the current state of this mega development.
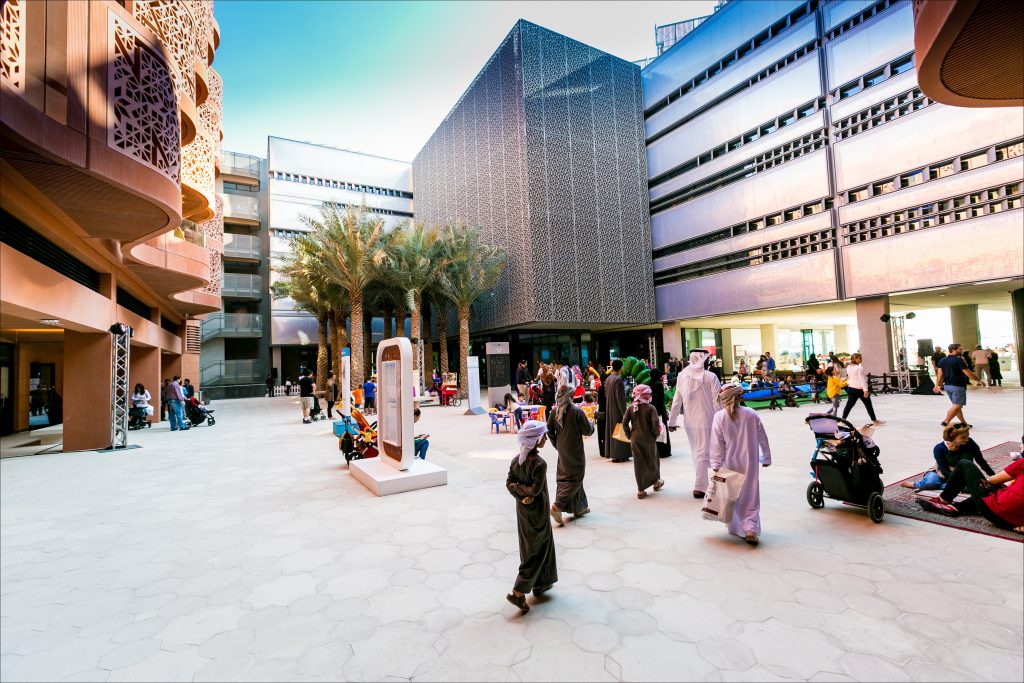
Wind Tower
In the centre of the city stands a 45m tall structure that helps in cooling down the city. Heat is a major issue for cities in the middle east. With the help of this tower and other features of the city of Masdar such as narrow and shaded streets, the city is roughly 10 degrees Celsius cooler than the streets of Abu Dhabi, some 17km away. The louvers on each side of the tower open and close automatically, based on the wind direction, and direct the wind down to the streets of the city. A piping system inside the tower mists treated grey water to cool down the air and create a natural air conditioning for the city.
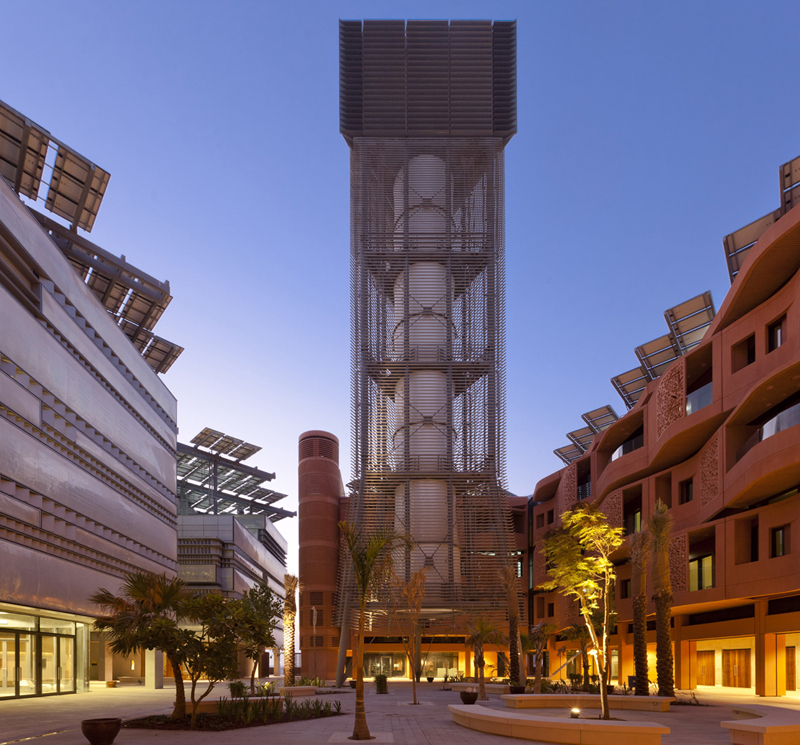
Shams 1 Concentrated Solar Plant
Shams 1 is a 2.5 square km facility utilizing 768 parabolic trough collectors that collect the heat from the sun and heat a water tube in the centre. The heat evaporates the water in the tube and the steam created will drive a turbine to generate electricity. The plant has a capacity of 100 MW and aims to generate power for Masdar and the city of Abu Dhabi. Experiments have been conducted to run this plant in conjunction with an absorption refrigerator (figure below) that would provide cooling for buildings in the city of Masdar.
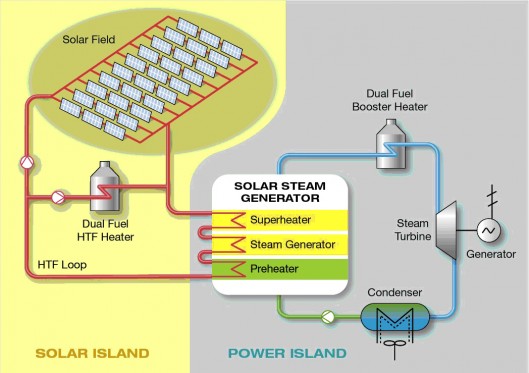
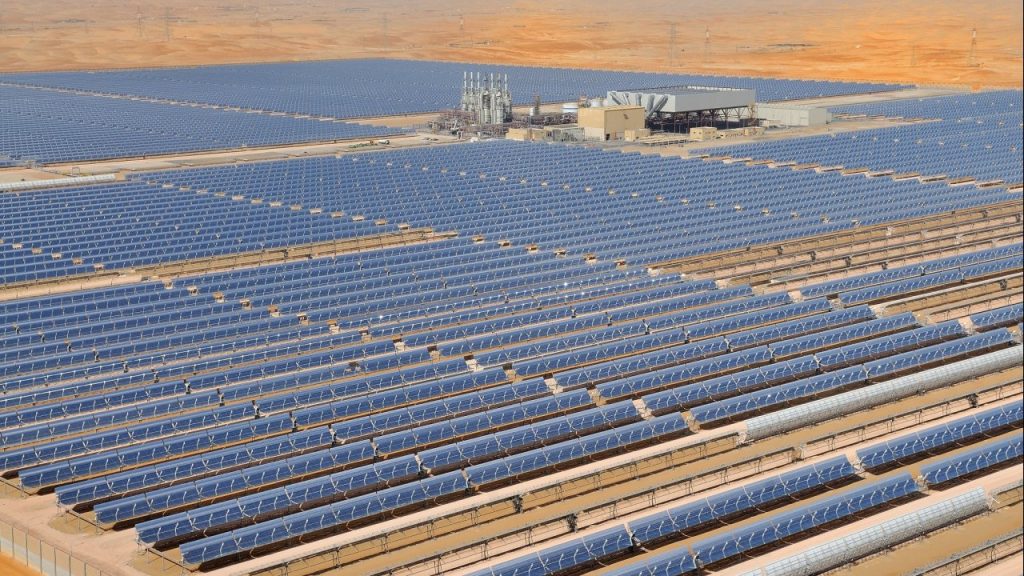
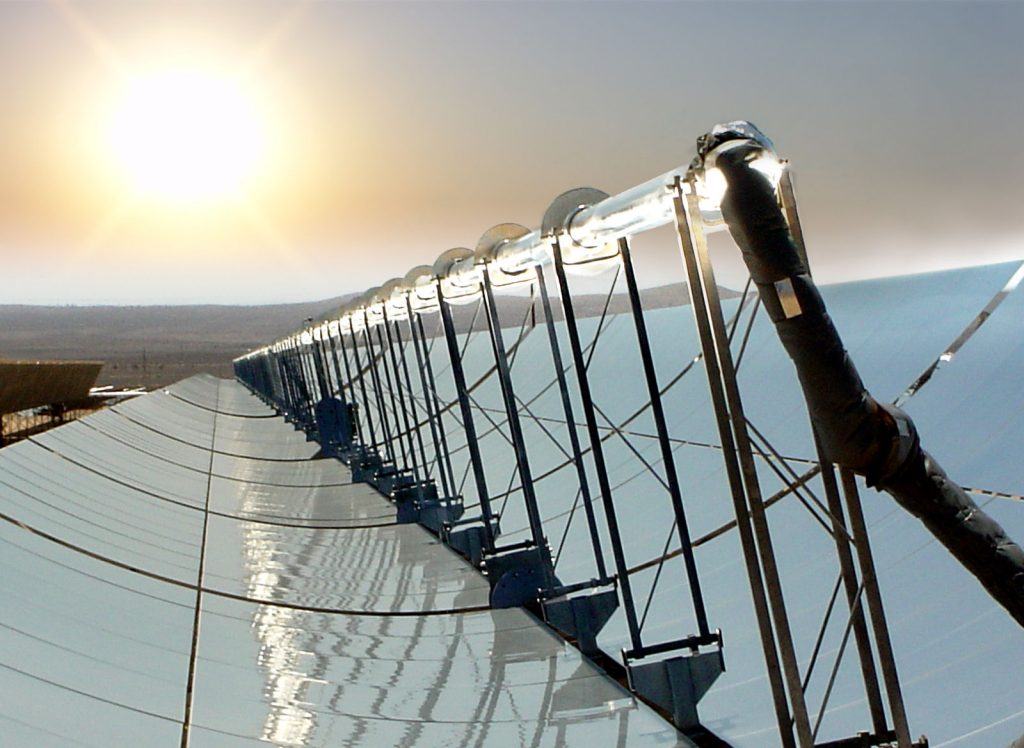
Solar PV Plant
Supplementing Shams 1, Masdar has a 0.21 square km photovoltaic plant that produces about 17,500 megawatt-hours of electricity annually with a 10 megawatt peak capacity. In addition to the solar plant, buildings in the city of Masdar have solar panels on the roof top, adding another 1 megawatt to the cities solar power capacity.
Personal Rapid Transit (PRT)
The overall layout of the city is designed to be pedestrian friendly and eliminate the need for cars. For travelling long distances in the city, planers designed a PRT system under the city that uses small electric vehicle to transport people to their destination. The vehicles are completely electric and operate without a driver underneath the city streets.
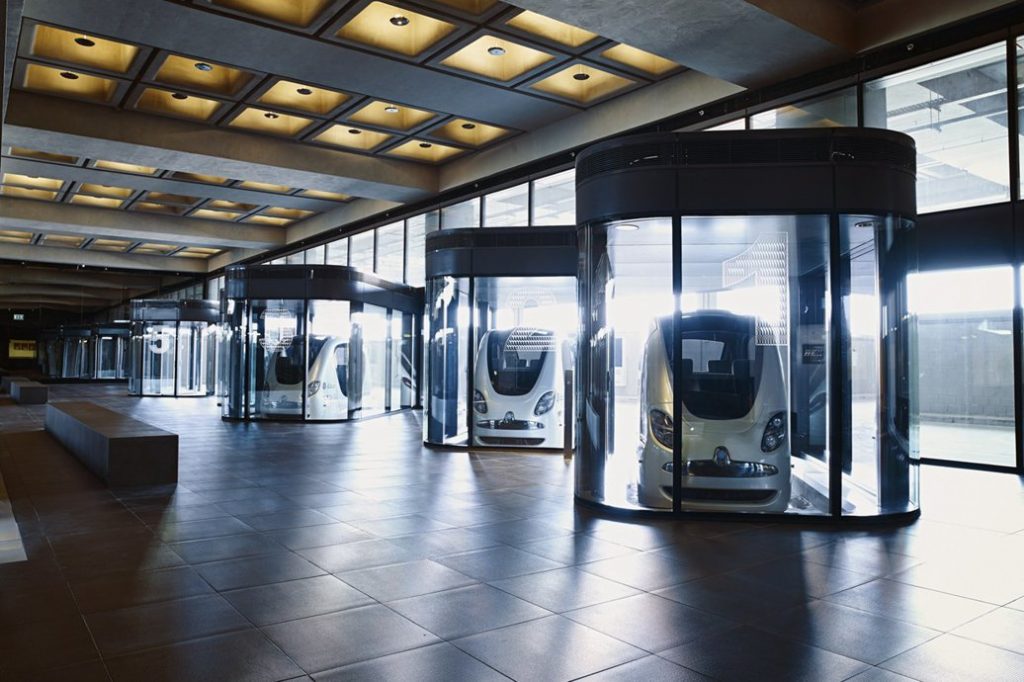
Geothermal Energy
Two 2.5 km deep wells were drilled to be used for thermal cooling and domestic hot water. One well is used for drawing hot water and the other for re-injecting the water after heat extraction. The heat extracted from the earth is sufficient for running an absorption refrigerator to create a cooling system for buildings, but the system has not been implemented yet.
Current State
The economic crisis of 2008 had a serious impact on implementing the plans for the city of Masdar. Investors were reluctant to invest in the city due to the uncertainty in the economy. Based on statics from 2016, only 2,000 people work in the city of Masdar and less than 300 people live in the city, who are all students at the Masdar Institute of Science and technology with free tuition and accommodation. The completion of the development is now pushed back to 2030.
The expensive PRT system has been completely scrapped. The system is no longer relevant because of the major improvements in the electric vehicle sector. The planners did not predict that electric vehicles, such as Tesla, would be developed and widely available in such short time.
The city has also moved away from being “zero-carbon”. The concept has been found very difficult to achieve. However, the projects in Masdar produce clean energy that is being exported to Abu Dhabi and reducing their footprint. This is where the issue of scale comes into place. Within the boundaries of Masdar, the city is not carbon free, but it is reducing the total carbon footprint of the UAE by providing clean energy to Abu Dhabi.
Masdar might have not been unsuccessful in creating a full scale city so far but, it has been a great opportunity for engineers and scientists to explore new areas and find solutions that would be used all around the world to reduce negative environmental impact. Masdar Institute is a hub for research and experimentation in urban planning and low-carbon infrastructure. The project also illustrates the forward thinking of planners and government officials who understand that the UAE cannot be dependent on fossil fuels for much longer.
This large-scale experiment could be further proof that we would benefit more by reducing the environmental impacts within existing cities rather than building brand new ones.
References
Youtube Video 1 by Fully Charged Show
Youtube Video 2 by Fully Charged Show
Figure 3 – Shams 1 Solar Plant Heat Exchange
Figure 4 – Shams 1 Concentrates Solar Plant