
A (gel) prototype we made in the lab
The original motivation of this work stems from the fabrication of hydrogel-based biomaterial by chemical patterning at the interfaces of two fluid streams in a parallel flow system that finds its application in the field of scaffold engineering. Scaffolds are biocompatible materials that act as a replacement to organ and tissue transplantation and designed to mimic the extracellular matrix by providing structural support that promotes cell attachment and proliferation. Natural polymers such as alginate or nano-fibrillated cellulose are particularly suit- able for in-situ layering as they gelate when contacted with a salt solution. Under creeping flow conditions, Boyd et al. demonstrated the potential of this process by printing sculpted bodies. They considered fabrication of the hydrogel at small scale by combining two laminar flows of the gel precursor solutions, a salt solution and an alginate solution, in a microfluidic device. We are motivated by the results of previous studies on microfluidic patterning of hydrogels and the scientific challenge to scale up the process to produce structures at larger scales.
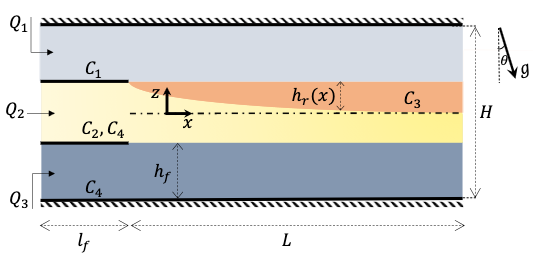
Overview of the experimental Hele-Shaw cell and variables. (a) Schematic of the Hele-Shaw cell at an inclination angle θ where Q1, Q2 and Q3 are the flowrates of each fluid stream. The upper layer includes salt solution, the middle layer is alginate and the bottom layer is water densified by sugar. As salt diffuses to the alginate, it reacts with the alginate and the gel layer forms. The position of the gel layer is defined as hr(x).
In this research, we consider scaling up the reactive multi-layer flow in a planar two-dimensional channel. In particular, we scale up the aspect ratio of the geometry. The inertial forces are small and the system operates at a relatively low Reynolds number, i.e. Re < 1. A particular application for this configuration is in the production of skin scaffolds. The skin scaffold is required to be thin and accurate in thickness. In this concept, this reactive multi-layered flow system in a Hele-Shaw cell can be considered as a good candidate for printing skin scaffolds. In this flow system, the gel layer develops on one interface due to the reaction between two fluid streams and the gelled product is lubricated and transported along the channel by the upper and lower Newtonian fluid streams (see Fig. 5.1(a)). What seems necessary here is first to maintain a stable flow configuration, and second to control the rate of the gel production under the stable flow regimes. We performed a systematic study to delineate the conditions for stable operation under different flow conditions and to determine the growth rate of the gel layer.
- Stability of multilayered flow:
we consider the unreactive and fully-reacted cases in order to study the possible flow regimes and instabilities that might occur under the effect of density differences at slight inclination angles. The experiments were performed for Re = 0.31 to characterise the operational stability as a function of inclination angle with no reaction. Our experimental observations confirm that the unreactive flows are unconditionally stable over the range of our experiments. Counterintuitively, the unconditional stability of the system fails in the case of a fully reacted flow when the middle layer is a gel. The surprising feature of our experiments is that hydrodynamically unstable flow regimes or mixing events were observed outside for the fully reacted case. It should be mentioned that these observations are in contrast with the numerical simulation of the problem that predicts unconditionally stable flow over the range of our experiments.
We observed buckling of the viscoplastic fluid where the inclination angle affects the resulting trajectory. We hypothesized that this complex behaviour can be attributed to the elasto-mechanical response of the Carbopol to the interfacial stress caused by the recirculating fluid in the upper and lower fluid stream (regimes I and III).

Representative diagrams of hydrodynamic stability of a fully reacted flow in a Hele-Shaw. (a) A representative stability diagram with complex behaviour. The data were collected with water in the upper layer (1), fully-reacted Carbopol in the middle layer (2), and a water-sugar mixture in the lower layer (3) (see Table 5.2, and Fig. 5.1). Note that Q1 = Q3 for all of the cases shown here. Regions I, II and III represent the three possible flow regimes that depend on the inclination angle and the hatched areas denote unstable flow regimes. For cases (I), (ii) and (iii) we display the time evolution of the second layer, measured at a fixed position, as a function of time where tmax is 130 s, 15 s and 25 s, respectively. (b) Representative fully developed velocity profiles for regions I–III in (a). Note that the three flow regimes (I–III) and corresponding velocity profiles were obtained from a 1-D theoretical prediction, while the unstable regions were found by experimental observations.
2. Growth rate of gel layer:
In the reactive process, the calcium salt diffuses from the top layer into the middle layer causing a gel transition at the interface. It should be noted that the time scale of the chemical reaction, t_ch is much shorter than hydrodynamic time scales of the problem that are the advection time, i.e. ta = H/Uc, diffusion time, i.e. tD = H2/D, and viscous time, i.e. tν = ρH2/μc, in this problem. The average diffusion coefficient of salt in liquid alginate is assumed to be roughly D ∼ 0.3 mm2/s [92, 107]. In addition, all our reactive experiments have been performed at the average velocity of 0.097 mm/s. Therefore, one can estimate tD ∼ 1700 s, ta ∼ 31 s, and tν ∼ 9 s, while the characteristic time scale at which reaction occurs is in the order of micro seconds. Hence, a clear separation of time scales of the problem is obtained, tch tν < ta < tD. Therefore, as the upper and middle fluids come into contact, the fast reaction instantaneously establishes a layer of reacted fluid at the interface with a yield stress and elevated viscosity. The fresh unreacted fluids are brought into contact at slow time scale of advection, and the reacted layer broadens diffusively at a slower time scale. In view of this, it can be assumed that the thickness of gel layer produced on the interface is dependent on the diffusive length scale of the reactive component, i.e. hr ∼ √taD. We performed a series of theoretical and experimental studies to examine the validity of this assumption.

(a) (b)Figure 5.6: Growth of the gel layer hr(x) with reaction in a Hele-Shaw cell: (a) Theoretical values obtained for the ratio of the thickness of the reacted layer over that of initial alginate layer before reaction, H0. The results were obtained for Da = 1000, and Pe =50, 100, 250, 500, 750, 1000. (b) Experimental results for the averaged thickness of the reacted layer normalized by the thickness of the channel versus the square root of the advective time scale over a diffusive time scale. The data reported in panel (b) correspond to Re = 0.31 (Q = 0.9 mL/min), 0.25% wt/wt-alginate, and 0.1% wt/wt-salt solution. Here, the thickness of the separation plate in the cell is presented by *.
MacKenzie J., Siren E., Daneshi M., Melnick R., Treskatis T., Wachs A., Kizhakkedathu J.N., Martinez D.M., Fibre-reinforced biocompatible hydrogel to replace single-use plastic tubing in the clinical setting. Chemical Engineering Journal. 2022 Jan 15;428:131786.