Mechanics and rheology of complex fluids
My research interests lie in fluid mechanics, with a focus on experimental studies of complex fluids in particular viscoplastic fluids (VPFs). A significant number of fluids that are categorized as VPFs: used in our daily life such as toothpaste, hand sanitizers, and peanut butter; in geophysical and industrial settings, such as magma, cement slurry, tailing fluids, and drilling mud. Studying the flow of complex fluids and non-colloidal suspensions have been the focus of my doctoral and current research activities.
As the manager of the complex fluids lab at UBC, I also organize training sessions and advise students in performing rheological tests and using rheo-imaging and rheo-scattering techniques.
My research studies are described as follows:
(I) Mechanics of bubbles rise in complex fluids.
The focus of this part of my research is on restoring polluted lakes. It is in collaboration with Professor Greg Lawrence at the Department of Civil Engineering, UBC.
Spatiotemporal images of first bubble propagation through three layers of Densified Carbopol (DC), glycerol, and Carbopol. Pure glycerol is used in cases (a)-(e). Glycerol concentration in case (f) is 80%. The concentration of Carbopol solutions used in DC and C layers are: a) DC=0.15%, C=0.06%; b) DC=0.15%, C=0.1%; c) DC=0.15%, C=0.2%; d) DC=0.18%, C=0.15%; e) DC=0.18%, C=0.1%; f) DC=0.15%, C=0.1%. As DC concentration increases, bubbles tend to have a larger volume. As glycerol concentration decreases, bubbles tend to have a less spherical shape. The DC layer in case (e) was dyed to investigate whether bubbles entrain the DC fluid into the glycerol layer.
The flow and trajectory of a rising bubble initially positioned within a yield stress fluid at a horizontal distance to a Newtonian layer. The panel on the left shows the numerical results and the experimental results are shown in the panel on the right.
The flow and trajectory of a bubble rising bubble in a viscous layer crossing a yield stress fluid at $\theta$= 72.5 . The panel on the left shows the numerical results and the experimental results are shown in the panel on the right.
(II) Multi-layer flows with yield stress fluids,
Evaluating the interface position and the shape of the interface between the fluids by solving the nonlinear wave equation obtained from the lubrication model:
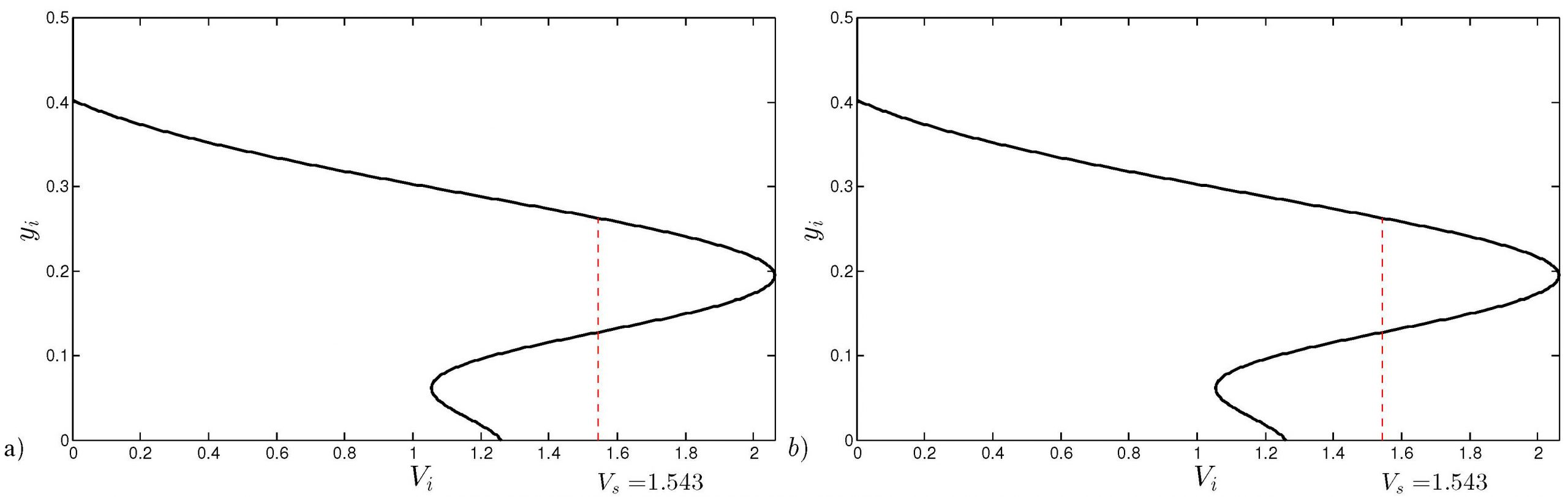
Interface propagation obtained from two-layer model: (a) Frontal shock; (b) contact shock (spike). M. Zare, et al., J. non-Newton. Fluid Mech, (2017), https://doi.org/10.1016/j.jnnfm.2017.06.002.
Computational results obtained for the density unstable displacement of a yield stress fluid from a channel:
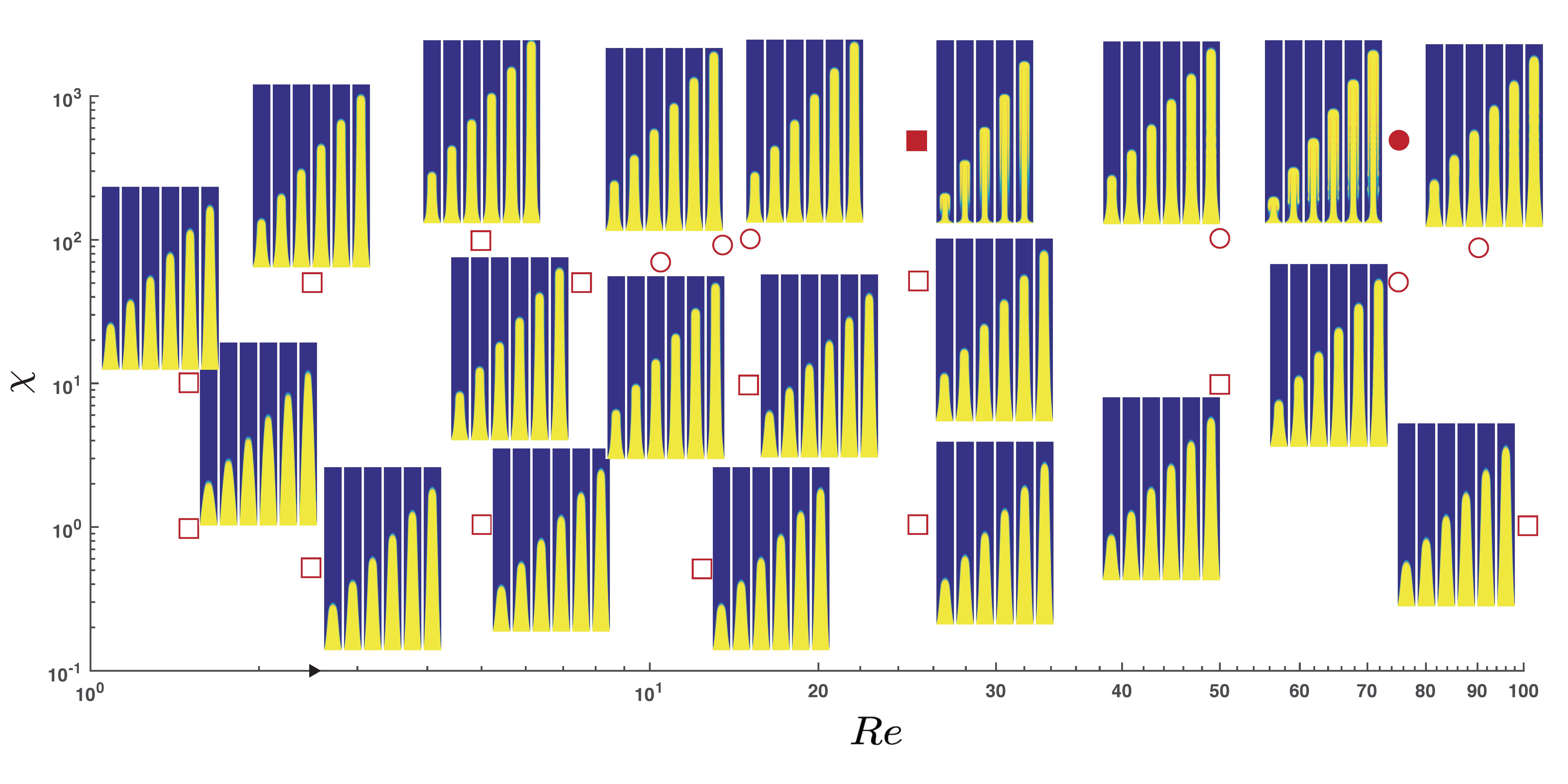
Panorama of flow types observed for Bingham (B)=1, and viscosity ratio (m)=3. Markers indicate data position in (Re, Re/Fr^2) plane with symbols indicating the corresponding flow regime [M. Zare, and I.A. Frigaard. J. non-Newton. Fluid Mech, (2018) https://doi.org/10.1016/j.jnnfm.2018.07.007].
(III) Onset of invasion of viscous fluids to a yield stress fluid column and their propagation through the gelled column,
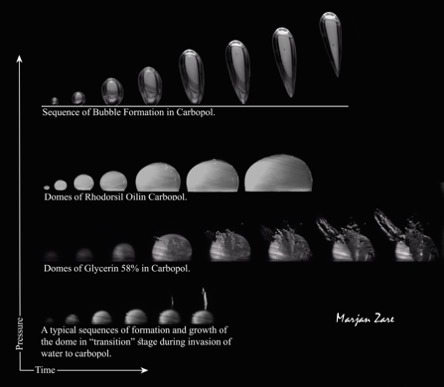
This figure shows the invasion process of air, rhodorsil oil, glycein, and water respectively from top to bottom to our model yield stress fluid, i.e carbopol [M. Zare, I. A. Frigaard, Phys. Fluids, https://doi.org/10.1063/1.5024718].