With pressures of climate change becoming a major global issue, the idea of resilient cities has become somewhat of a buzzword. I would like to focus on one overarching theme in resilient city literature and solutions: flexibility. 100 Resilient Cities defines urban resilience as “the capacity of individuals, communities, institutions, businesses, and systems within a city to survive, adapt, and grow no matter what kinds of chronic stresses and acute shocks they experience.” This is achieved “By strengthening the underlying fabric of a city and better understanding the potential shocks and stresses it may face.”
Change is inevitable, so our cities must be able to absorb impacts, react and adapt accordingly. However, infrastructure is usually something seen as permanent and lasting (de Haan). In mechanics, one learns that brittle fracture is much more dangerous than ductile fracture. It acts as a warning of the damage to come, and can absorb more energy prior to fracture, resulting in a stronger and more resilient structure. Why not apply this at a city scale?
In general, flexibility means the possibility to introduce certain options with the assumption of changing configuration of system parameters or system components in time (Kośmieja and Pasławski). De Haan points out that “the complexity of, especially nowadays, infrastructure systems suggests that we step away from attempts to control circumstances and prepare for their consequences.” My interpretation of flexible infrastructure lies in understanding that there are different possible outcomes and acknowledging that cites (and environments) change.
Flexibility can come in different scales. For example, buildings can be designed to be more seismically sound by including literal flexible materials within them, such as timber. This can be seen in Tūranga, the new central library in Christchurch, New Zealand, designed by Schmidt Hammer Lassen of Denmark. The building includes a “seismic force-resisting system [that] is made up of a series of massive concrete walls that can rock and shift to isolate the building from peak accelerations during an earthquake.” Along with the use of pre-tensioned steel cables that stretch and flex, allowing the building to right-itself in the event of swaying, this structure is virtually earthquake-proof.
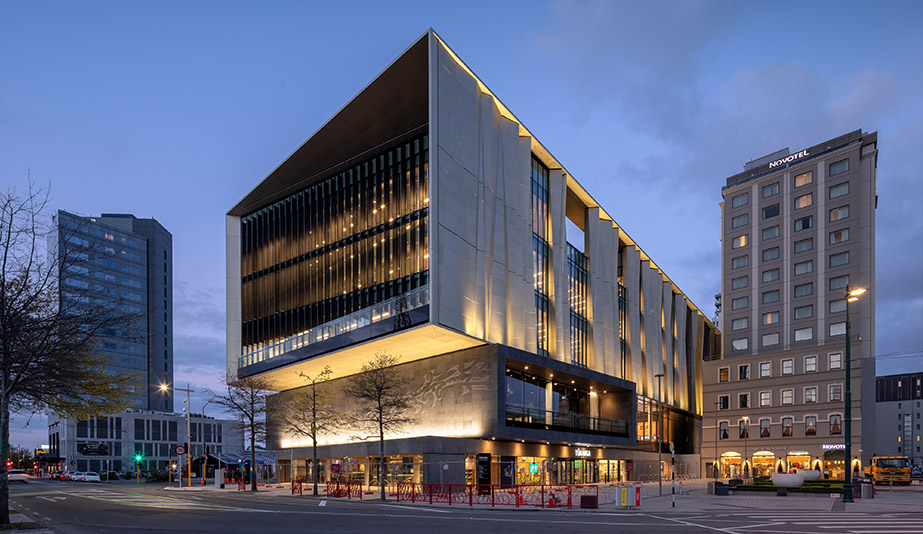
At a larger scale, the Østerbro neighbourhood of Copenhagen is a resilient neighbourhood that incorporates flexibility in rainfall systems. Due to climate change, Copenhagen has dealt with increasing levels of high-intensity rainfall that original systems could not cope with. In the creating of resilient infrastructure, these increased rainwater levels were seen as an opportunity, rather than an issue that needed to be removed. As the old rainwater management systems could not be changed (an example of the rigidity of non-resilient infrastructure), and due to minimal space restrictions, new innovations needed to be implemented locally and in tandem with increases in public green spaces. For example, in Tåsinge Plads, a square in the community, rainwater is diverted away from roofs and squares to keep the water out of sewers, while the storm water is collected in green urban areas to support the incorporation of wild urban nature in the community. In the few paved areas, ‘water parasols’ were created for children as play elements, that double as catchment basins that pump water through small channels to green spots (these are the inverted umbrella-like black structures in the image below). Here, it is important to see that flexibility is not just physical, it is a mindset – and one must bring a systems thinking approach to planning for flexibility.

One of my favourite examples of resilient infrastructure can be seen in Rotterdam. Similar water issues are being dealt with here, where water squares have been created to act as social spaces, but in the event of flooding, can also hold excess water. The flexibility in this site is clear, with multiple functions that addresses urban social living along with sustainable solutions simply, without the need for any advanced technical solutions or materials.
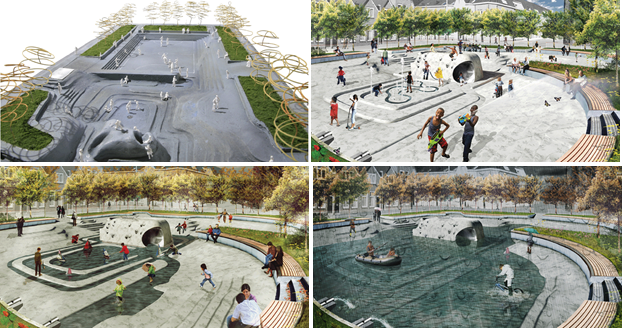
Urban resiliency is a buzzword for a reason: it is vital that cities address issues of our changing environments immediately, as well as do what is possible to prevent further global environmental degradation. A key component to this change is to introduce flexibility in approaching problems and at different scales. The Anthropocene is upon us, human activity is indeed the strongest geo-technical force at this moment, but why not try and make this impact a positive one?
OTHER RESOURCES
Maria Kośmieja, Jerzy Pasławski – https://doi.org/10.1016/j.proeng.2015.10.013
de Haan – https://doi.org/10.1016/j.futures.2011.06.001
http://www.rotterdamclimateinitiative.nl/documents/2015- enouder/Documenten/20121210_RAS_EN_lr_versie_4.pdf
https://www.sciencedirect.com/science/article/pii/S0016328711001352
https://link.springer.com/content/pdf/10.1007%2F978-3-319-49730-3.pdf
https://www.curbed.com/2018/5/11/17346550/organic-architecture-infrastructure-green-design
https://www.worldbank.org/en/results/2017/12/01/resilient-cities
https://openknowledge.worldbank.org/handle/10986/11986
https://www.resilientcity.org/index.cfm?id=11900
https://www.iiste.org/Journals/index.php/CER/article/viewFile/38207/39282
http://www.100resilientcities.org/resources/