There are a variety of ways to classify and explain the causes of landslides. Some authors may differentiate between risk factors and triggers, while others might classify landslide causes depending on their origin: morphological, geological, physical, human etc. For the sake of simplicity, it can be generalized that all causes of landslides act in two main ways: (a) by increasing shear stress, and, conversely, (b) by reducing the shear strength of the slope. 1
When the shear stresses exceed the resistance to shearing of the materials that form the slope, a landslide will be triggered.
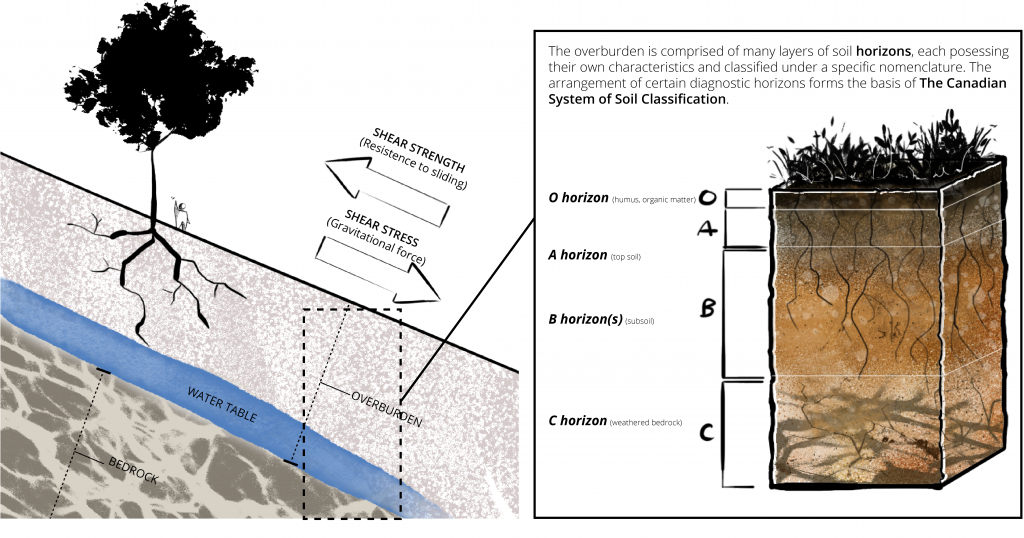
In addition to increasing shear stress or reducing shear strength, the causes can also be viewed as:
- Inherent to the material or stemming from its initial characteristics or state. These causes are part of the geologic setting of the slope that may be favorable to landslides, exhibit little or no change during the useful life of a structure, and may exist for a long period of time without failure (examples include the soil composition of a slope, the soil’s structure, and the slope’s geometry).
- External or ‘variable’ (e.g., addition or removal of vegetation, geologic shocks such as earthquakes, frost wedging, etc.).
- Obviously, few (if any) landslides are explained by only one risk factor, cause, or trigger. Moreover, certain individual events can simultaneously reduce the shear force and increase the shear stress: a flash flood, for example, adds weight to the soil (increases shear) while lubricating colloidal soil particles (reduces force). §
Factors that increase shear stress(es)
These factors account for most of the structural soil instabilities leading to landslides. Increases in shear stresses (or, in other words, an increase of the pull of gravity) are often a consequence of:
- Slope undercutting by removal of lateral support, such as the one caused by the erosive action of water or glaciers, for example.
- Surcharge, which may derive from both natural 1 or human 2 agencies.
- “Transitory” earth stresses caused by earthquakes or volcanism, or by human interventions such as blasting, traffic and machinery, quarries, removal of support structures, creation of ponds and lakes, etc.
- Lateral pressure exerted by frost wedging and heaving.
- Wind stress (more specifically through the lateral pressure of swaying trees).
- Weight of rain, hail, snow, and water from springs, accumulation de talus, Seepage pressures of percolating water, etc.
- Construction of fill, stockpiles of ore or rock, waste piles, weight of buildings and other structures, weight of water from leaking pipelines, sewers, canals, and reservoirs, etc.
Factors that reduce shear strength
The shear strength of a slope is mainly dependent on the cohesiveness of the materials comprising either “the overburden” or “the bedrock” (further defined below).
The main forces involved in maintaining this cohesive strength are: (a) the ability of particles to stick or adhere together, (b) the frictional resistance between the groundmass and the sliding surface, and (c) the resistive force generated by the anchoring action of tree roots. Inversely, some of the factors that contribute to decrease shear strength are:
- The presence of zones of weakness in overburden or underlying bedrock produced by bedding surfaces, (fractures and joints, layers of weaker soils such as of silt and clay in the ground mass, etc.)
- Undercutting by the removal of downslope support of the mass, commonly by stream cutting or roads
- The development of a temporary water table 1 above a potential failure surface ; and
- The progressive soil creep, which happens slowly over time and produces strain-dependent drop in strength within the slope.
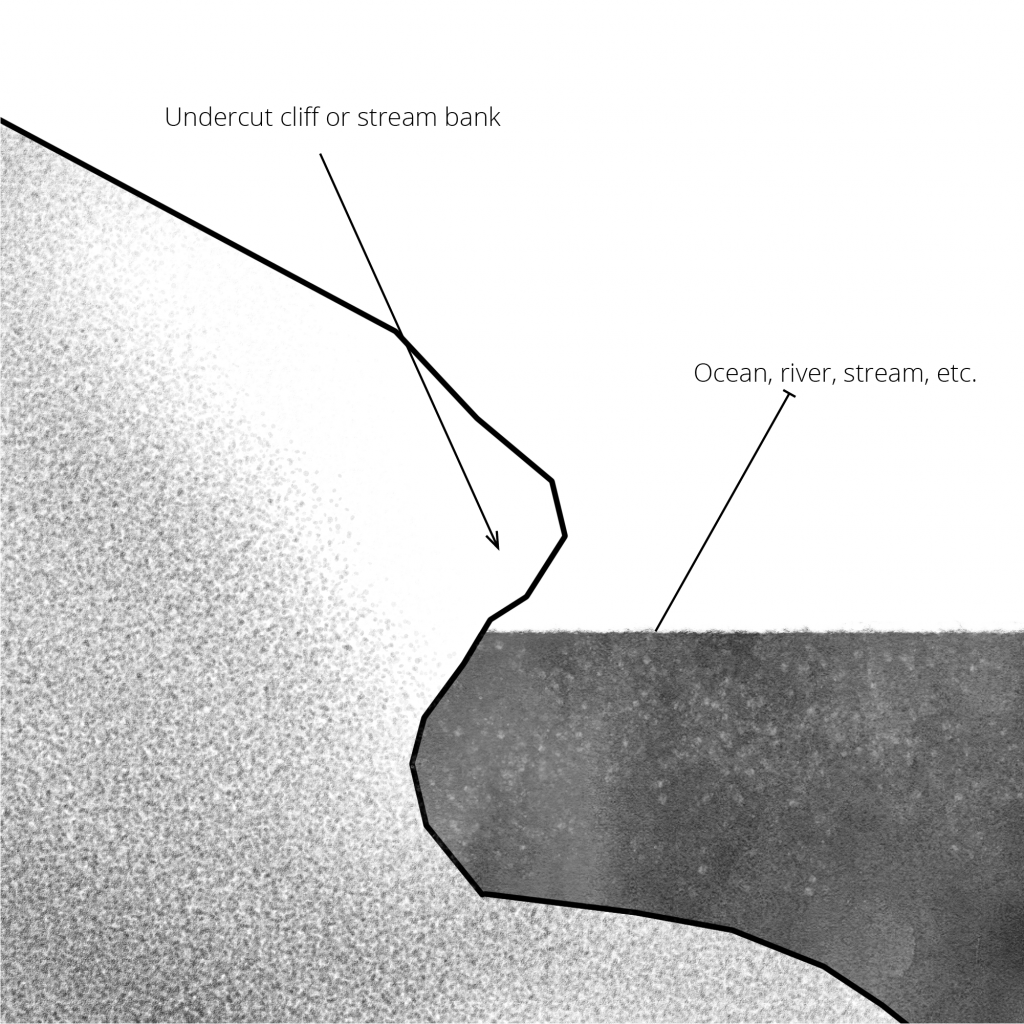
- See the section “Influence of hydrologic conditions” below.
Beyond a general understanding of how increases in shear stress or decreases in shear strength can induce slope failures, it can be interesting for the landscape architect to know the influence of certain parameters on slope stability. These are obviously much more numerous and complex than what is discussed here, but a brief overview on the topic can serve as a starting point for the design professional who might be interested in mitigating such natural hazards through design.
It is self-evident that an understanding of the general concepts presented below does not and cannot replace a thorough study of a representative soil profile 1 in situ prior to any design intervention by a qualified professional. Professional designers, however, ought to actively participate in that process, as it will undoubtably reveal crucial information and infer a variety of design considerations and choices.
- A soil profile is a vertical cross-section of the soil, usually realized in order to analyze, study or assess its different horizons, their depth, order, etc.
Influence of geological conditions
As mentioned in the previous section, the physical properties of soils are a foremost factor in slope stability. Some soils are weaker than others, thus breaking more easily when they come under stress resulting in slope failure.
When discussing landslides, experts often divide the soil mass in two components: the overburden and the bedrock. The former term, which derives from the mining industry, refers to the material that lies above the area that lends itself to economical exploitation; in the case of landslides, it is, simply put, any soil material resting over the bedrock. Though not very useful from a pedological perspective (as it encompasses a whole amalgam of different soil horizons 1, which will vary considerably in terms of textural, compositional, and chemical properties even at the scale of a single site), this terminology allows for a simpler understanding of the physical mechanisms underlying mass movements as a whole and will therefore be used in this blog.
- Overburden materials
A basic concept seen in the previous section states that soil cohesiveness is one of the most crucial parameters in determining landslide susceptibility, particularly in the case of debris avalanches and debris flows. The cohesiveness of the overburden materials is largely determined by clay mineralogy and moisture content. Generally speaking, clayey materials do possess high shear strength, cohesion, and angle of internal friction 2; but only when dry. When wet, these particles mobilize quickly and, ultimately, can ‘deform’ in presence of water excess, which leads to slope failure.
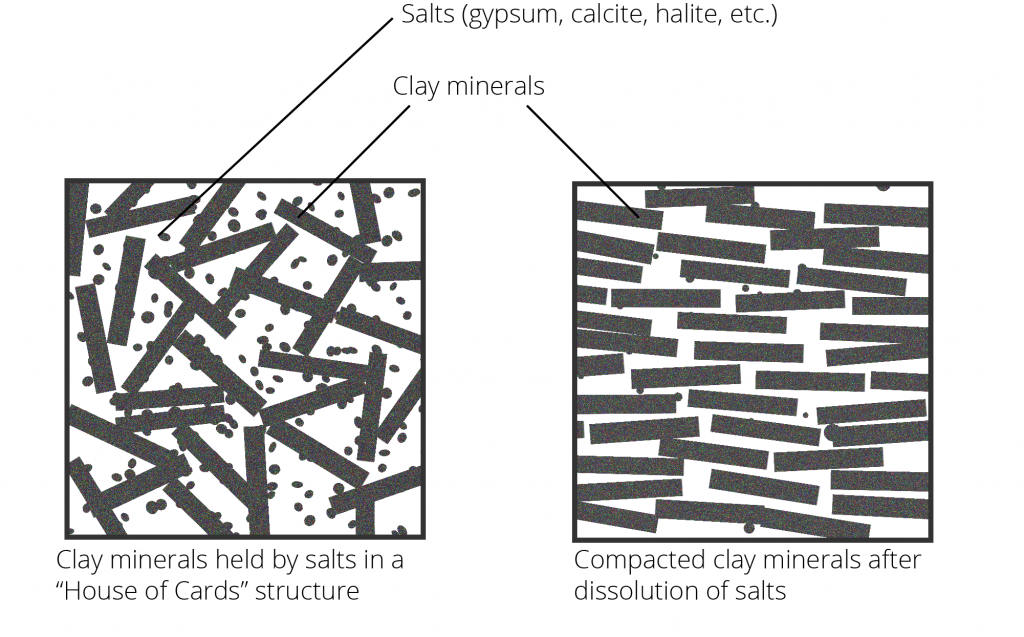
- Bedrock
It is self-evident that the easier certain soil types can be weathered, the more prone they are to landslides: weathered materials form fissures and gaps, in which water can penetrate, loosening the particles and reducing the soil’s shear strength. The constitution of the bedrock does not only indicate landslide susceptibility, it can also reveal the type of mass movement that is most likely to occur.
For example, materials more susceptible to weathering (such as medium- to fine grained sedimentary rocks) develop a high degree of cohesion and mobility and are prone to slope movements of the creep, slump and earthflow types. Slopes underlain by hard and dense sedimentary and metamorphic rocks are likely to develop an overburden that’s coarse-sized and low in clay-sized particles, which results in slope movements of the debris avalanche and debris flow types. Fine-grained andesite and basalt flow rock also develop thin, coarse-textured overburden on steep surfaces, with resultant debris avalanche and debris flow failures.
The bedrock’s internal structure may also have a significant influence on slope stability. Highly jointed or fractured bedrock slopes provide little mechanical support to overlying materials and create avenues for concentrated subsurface water movement, while also providing avenues for deep penetration of surface and ground water.
- Topography
At a larger scale, a summary analysis of certain topographic patterns can help inferring certain areas at greater risk for mass movements within a distinct landscape unit.
Shallow, linear depressions (or “swales”) on hillslopes are common points of origin for debris avalanches and debris flows, as they are usually created by the weathering of bedrock along zones of weakness. The converging flows of groundwater into these depressions during periods of storm precipitation or rapid snowmelt can cause the buildup of temporary perched water tables, which can lead to lateral slope undercutting and failure due to pore-water pressure or deformation of the deeper soil layer.
The presence of deep gullies and canyons along the slope are also problematic, as they can act as debris collectors (either from adjacent hillslopes or from their own banks) and accumulate soil, rock and organic matter into their channels. If the quantities of these debris are important enough and are not normally evacuated, temporary dams may develop, which can induce different types of debris flows or other mass movements during storm events.
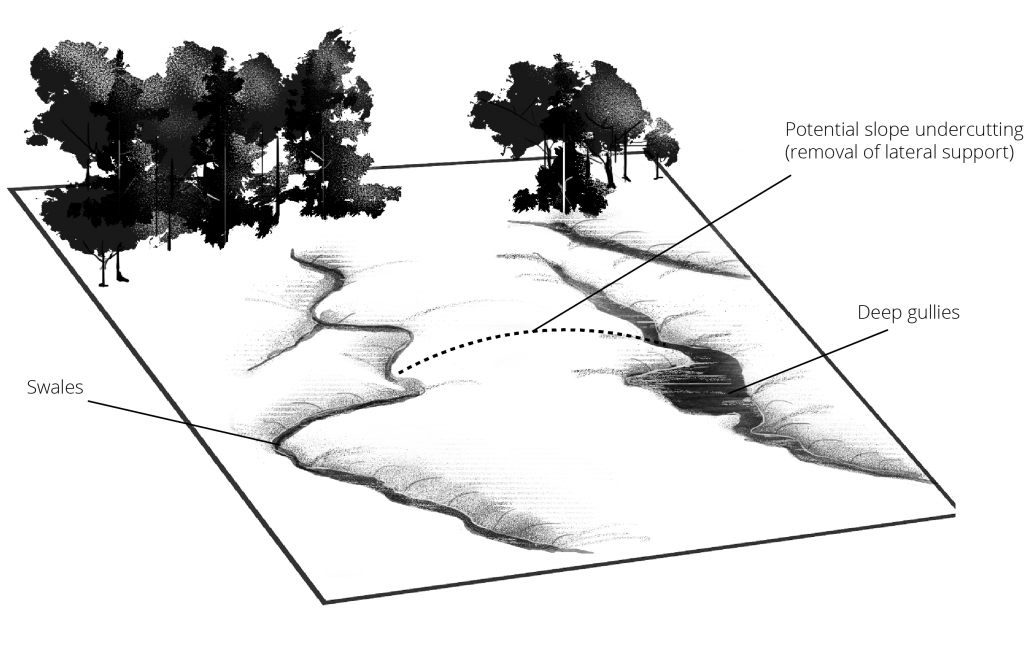
- A soil horizon is a layer parallel to the soil surface whose physical, chemical and biological characteristics differ from the layers above and beneath. They are widely used for soil classification, and a typical cross-section of a soil will generally reveal multiple horizons. §
- A measure of the ability of a unit of rock or soil to withstand a shear stress. §
Influence of vegetation
Tree cover and vegetation can influence slope stability and landslide occurrence in a number of ways. Generally speaking, both affect:
- The amount and intensity of rainfall reaching the surface of the soil (interception),
- The amount of water stored in the overburden (through evapotranspiration), and,
- The strength developed along a potential failure surface.
The first point (interception) refers to the phenomenon where a dense tree and vegetation canopy can, to some extent, intercept some of the water from the rain and prevent it to reach the soil, therefore reducing the lubrification of soil particles. However, in areas of high total rainfall or during large storms (which is when most slope movements occur), the direct effect of interception on the water budget in the overburden material is probably not very large.
The effect of evapotranspiration is probably more significant in stabilizing slopes, especially in areas of low rainfall, as it keeps the groundwater table at greater depths and avoids lubrication of soil particles on the surface potentially leading to slope failure. In areas characterized by warm, dry summers, evapotranspiration is particularly useful in reducing soil saturation resulting from the first storms of the fall; in areas of continuous high rainfall (like in B.C.) or those with arid or semi-arid climates, however, evapotranspirational withdrawals are probably negligible during the time period when most landslides occur. Moreover, when the root mass is shallow in the soil and recharge water rapidly, the added weight of the trees can even contribute to slope failures in major storm events.
The strongest influence of tree cover is through the root system of trees and other vegetation, which may increase the shear strength of unstable overburden by (a) anchoring through the mass into fractures in bedrock, (b) providing continuous long-fiber binders within the overburden (a fiber reinforcing effect) and (c) tying the slope together across zones of weakness or instability.
The synergy of these actions can be significant in reducing landslide risks; in some extremely steep areas, root anchoring alone may be the dominant factor in maintaining slope equilibrium in an otherwise unstable area.
Influence of hydrologic conditions
Slope saturation by water is one of the most crucial and primary phenomena that leads to slope failure. Water does not only contribute to the gravitational pull (shear stress) as it gets absorbed into the soil and adds weight to it; it also decreases the soil cohesiveness (shear strength) by forcing the soil particles apart and increasing the pore pressure within the soil.
Water erosion may also be a major trigger for landslides, as the removal of cementing material decreases cohesion of rocks and saps some of a slope’s strength, preparing it for failure by mass movement. On the other hand, more moderate amounts of water can help preventing slope failures. When water does not completely fill the pore spaces between the grains of soil, it creates a film around each grain, which can cause loose soil grains and particles to stick to each other due to surface tension. A water content below the saturation point may thus contribute to an increase in shear strength.
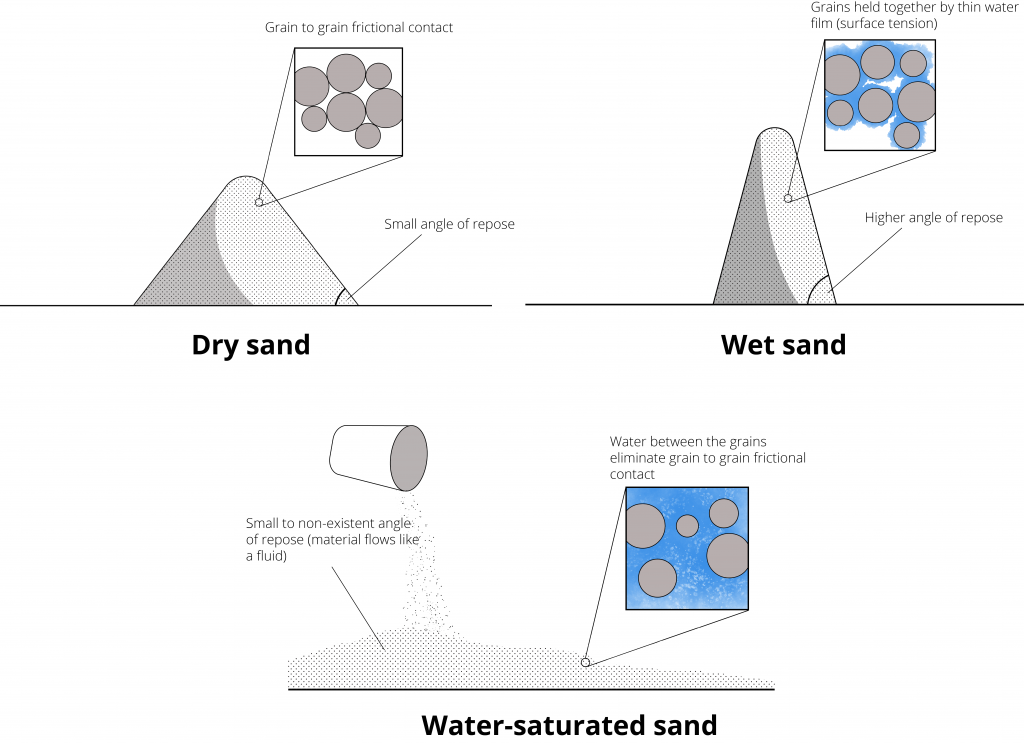