Parisa Mohammadi | MEL Candidate | Dec 17, 2021.
Mentor: Mark Goudsblom, University of the Fraser Valley
Executive Summary
There is an increasing need and obligation to reduce British Columbia’s Greenhouse gas emissions in the foreseeable future. The University of the Fraser Valley (UFV) aims to invest in renewable energy technologies and be a regional leader in sustainable development to stay on track with the provincial government energy policies.
In March 2021, a solar photovoltaic (PV) project is implemented to provide energy for Building G (Library) of UFV Abbotsford Campus. The project’s primary goals were to provide shelter from electrical rate increases, generate electricity from a reliable source, lower annual operating costs, and decrease GHG emissions.
This capstone explores to what extent the current PV system successfully met its primary goals and what considerations should be taken into account for future sites. The three main pillars of sustainability will be covered in this research to understand the effectiveness obtained with the renewable energy.
Goal and Objectives
This study aims to explore and compare the effectiveness obtained with solar energy. The project investigates to what extent the current PV system successfully met its primary goals and what factors should be considered for future sites. The following subjects will be discussed throughout this report:
- Collect data and analyze energy supply and demand
- Conduct financial analysis of the proposed system (NPV, payback period, and other economic indicators)
- Carry out performance Measurement and Verification (M&V) analysis
- Calculate energy performance indicators according to the BC building code
- Calculate saved GHG Emissions
- Forecast energy saving and GHG emissions for the project lifecycle
- Analyze the impact of extreme weather events on the performance of the system
- Provide recommendations on improving energy efficiency and reliability of future projects
Data Collection
The main sources of data that have been used in this research are:
- Energy Consumption: Monthly Natural Gas and Electricity Consumption (UFV Billing Report)
- Electricity Production: Solar Edge Real-time dashboard (Daily Production)
- Weather Data: NASA Climate Data
- Air quality and meteorological Data: BC Air Data Archive
Information on the electricity and gas bill for four years, starting from April 2017 to October 2021, is collected from UFV monthly consumption reports. The information gotten includes the billing period, natural gas consumption (GJ), electricity consumption (kWh), electricity cost (CAD), natural gas cost (CAD), electricity and natural gas emissions (tCO2).
To account for the effect of weather conditions on the gas consumption the Heating Degree Days (HDDs) values for the location were considered as the factor of influence. This was gotten from the NASA database through the RETScreen software for the same period as the energy bill. All other weather parameter data like the average air temperature, the daily solar radiation, relative humidity, precipitation, earth temperature were also obtained from NASA for the same period. Air quality and meteorological data obtained from the BC air data archive are also used for environmental analysis.
Data Preparation
Data preparation is an important step after the data collection. To do this, the below steps are followed:
- Download electricity production data from Solar Edge Dashboard
- Identifying missing data
- Excluding data due to the system alerts
- Finally, 80% of the initial data is considered as valid data for the next phase (data analysis)
After the data preparation and all other necessary information needed for analysis, the next stage is the data preparation using RETScreen Expert software. Through the software, the weather information gotten from the building includes; the latitude and longitude, the climate zone, the building elevation, the heating and the cooling design temperature, and the earth temperature amplitude. This was collected for both the climate data location and the facility location.
Data Analysis
After the collection, harmonization, and standardization of all the data, the last stage is the establishment of a relationship using the information inputted in the software. A graph was created to visualize the data qualitatively for this to be achieved. This was immediately followed by establishing time series and line graphs using the regression analysis baseline. And this eventually leads to setting up the cumulative sum (CUSUM) for the energy savings, development of a controlled chat, and the establishment of the M&V graph.
To effectively determine the net energy savings for this research, the regression analysis, CUSUM, and M&V were separately established for the whole data set, the period before the energy efficiency measure implantation, and the period after the efficiency measure implication.
After excluding missing and invalid data, 80% of the initial data is considers as valid data and used to data analysis.
Energy Performance of the Building
Energy Consumption Analysis
The first step is importing four years of natural gas consumption data into the software; this was followed by calculating the fuel consumption per day (GJ/day). After that, NASA satellite weather data were downloaded for the same period. Figure 1 shows the monthly electricity and natural gas consumption in the understudied building from April 2017 to October 2021.
Total Energy Consumption: 1’482 MWh/year
Energy Consumption: 280 kWh/m2/yr
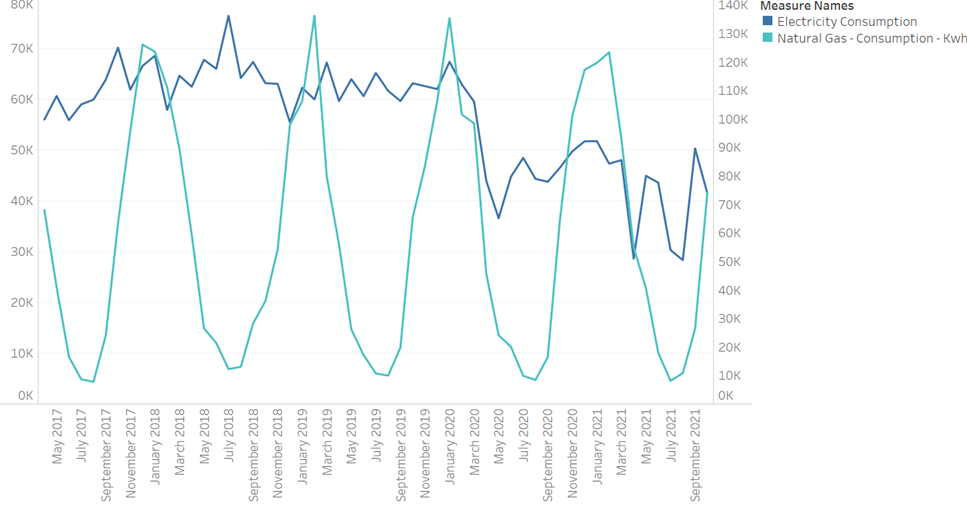
Baseline Model
The baseline is the period before the implementation of energy efficiency measures. In this case, the energy efficiency measure is a solar PV system installed in April 2021.
Despite the availability of consumption energy data for the four years (2017-2020), the baseline data is limited to 2 years: April 2017 to March 2019. The reason is that the university was closed for several months in 2020 and 2021, so the occupants in the library building were less than the usual time. Therefore, the data for the years 2020 and 2021 have been excluded from baseline analysis:
- Baseline Data: April 2017 to March 2019 (2 years)
- Excluded Data: April 2020 to March 2021 (Buidilge closure due to COVID-19 pandemic)
Baseline analysis shows that before the energy conservation measure, about 49% of the total energy came from natural gas and 51% from the grid. Figure 8 shows the ratio of different energy sources before and after the ECM.
After installing the solar PV system, the electricity produced by solar PVs has been replaced by electricity from the grid. In the proposed case, about 9% of the total energy required by building G would be supplied by solar energy, while electricity from the grid will contribute to 42%. Also, the share of the natural gas ratio would remain constant.
The baseline energy analysis shows that the total energy consumption is 1’482 MWh/year, equivalent to 280 kWh/m2/year.
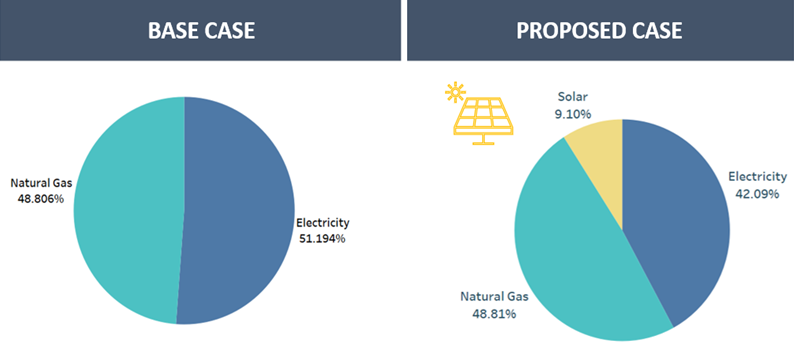
The analysis of the energy consumption shows that there is a direct correlation between natural gas consumption and heating degree days (HDD). The amount of natural gas is the dependent variable in the regression equation, and HDD is the independent variable. Figure 3 shows the relationship between these variables.
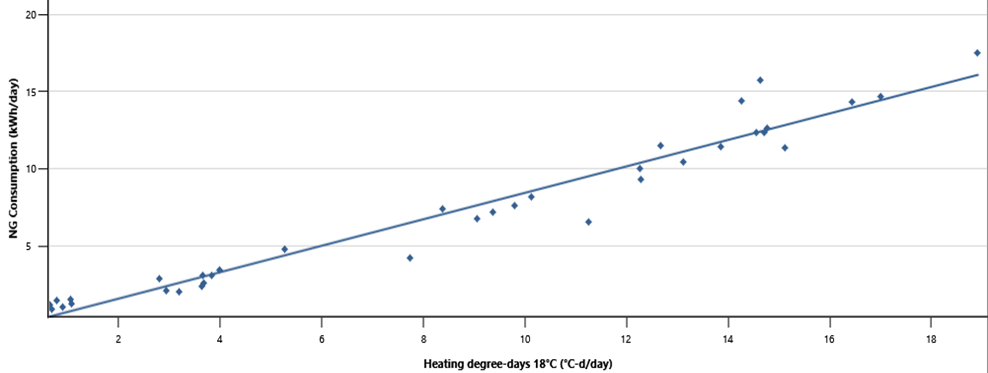
In addition, r-squared measures how well a linear regression model fits the data. In this case, R2=0.95 (which is close to 1) indicates a positive linear correlation between the two variables, natural gas consumption and HDD. The R2 value for the regression equation is calculated based on the fiscal year 2017-2019, which was the period before the implementation of the energy efficiency measure.
Regression Equation: Y= 0.8578 * X – 0.1494 & R2= 0.9506
The analysis of the energy consumption shows that there is no correlation between electricity consumption and heating degree days (HDD), as shown in figure 4. In this case, electricity consumption is the dependent variable, and HDD is the independent variable.
Regression Equation: Y= – 0.3407 * X – 0.2069 & R2= 0.0002
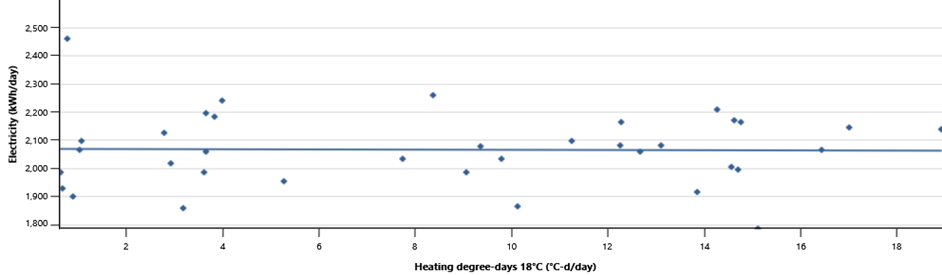
Performance Metrics (TEDI, TEUI, GHGI)
The BC building code has identified some indicators to provide a benchmark for energy performance improvement in buildings. These indicators include Thermal Energy Demand Intensity (TEDI), Total Energy Use Intensity (TEUI), and Green-House Gasses Intensity (GHGI). These metrics are defined as:
- Thermal Energy Demand Intensity (TEDI) provides a measure of the amount of energy a building requires to maintain an indoor temperature that is thermally comfortable for occupants, per meter of conditioned floor area per year.
- Total Energy Use Intensity (TEUI) provides a measure of a building’s total energy use, including both “process” and “regulated” loads, per meter of building area per year.
- Greenhouse Gasses Intensity (GHGI) is the total greenhouse gas emissions associated with using all energy utilities on site.
CUSUM Analysis
The cumulative sum control chart is a powerful technique for illustrating the energy performance of an energy-consuming system. CUSUM is the cumulative sum of the difference between the actual consumption and the baseline.
Figure 5 shows the CUSUM graph of the whole dataset. The flat part of the CUSUM line indicates that the consumption baseline has no change cumulatively when compared with itself, as would be expected. The downward trend after PV installation shows that energy is being saved because of the renewable source of energy. The energy consumption drop before installing a solar photovoltaic system is related to the campus closure due to the Covid pandemic starting in 2020.
Total energy saved resulting from ECM is estimated to be 167 MWh for seven months, from April to October 2021.
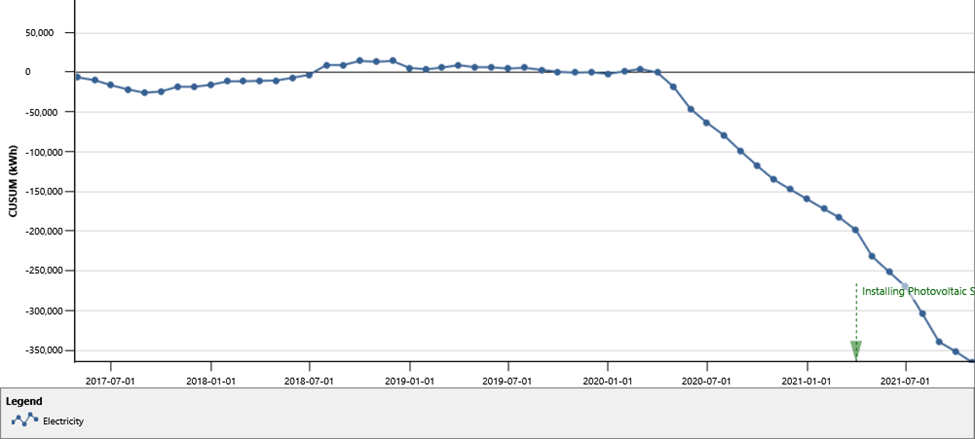
Measurement and Verification (M&V)
M&V is the process of planning, measuring, collecting, and analyzing data to verify and report energy savings within an individual facility resulting from the implementation of ECMs. As discussed earlier, the introduction of renewable energy technologies is a way of improving energy performance in an existing building. This is done by using a renewable energy source that aims at improving and optimizing the energy performance in a building. Hence, an energy-saving measure based on building energy consumption is needed to derive proper energy reduction and to optimize the cost of investment by carrying out the Measurement and Verification (M&V) analysis. M&V graph in figure 6 clearly shows the energy savings after ECM implementation.

Energy Production Forecast
Energy savings can only be detected by comparing the energy consumed before and after project implementation. This is done because energy saved during project execution cannot be measured directly since they constitute the absence of energy consumed. Energy saving is calculated to be 167 MWh for seven months from April to October 2021. In this case, saved energy is more than the system’s annual production (>135MWh). The reason is that in 2021some classes have been hybrid or just online, which affects the number of students who use library facilities and is different from the time when all students were attending classes in person. In other words, the Abbotsford campus in 2021 has not yet fully returned to the pre-pandemic period (2017-2019).
If we consider that this trend continues for the next five months (November 2021 to March 2022), then:
- Forecast Energy Saving: 190 MWh (12 months)
- Forecast Cost Saving: 190MWh * 0.097 $/kWh= 18’430$

Financial Analysis
The economic viability of the solar PV system at building G is assessed based on net present values (NPV), annual lifecycle savings (ALCS), cumulative cash flows, and simple payback and equity payback periods. Assumptions are as below:
- Initial costs and parameters for financial analysis are summarized in the report. The other assumption is as follows: The incentive, or grant, the amount is calculated as 80% of the total initial costs.
- The fiscal year starts on April
- The O&M costs for photovoltaic systems include an annual contribution for an inverter replacement
The analysis shows that the total annual costs and the annual life cycle savings would be CAD 10,698 and 11752 CAD/year, respectively. Additionally, the payback periods (simple and equity) give general indications of how soon investments in the PV project will start yielding profits. It means that projects with shorter payback periods will be more desirable. The values of the simple and equity payback periods are determined as 3.6 and 3.4 years, respectively. The cumulative cash flows graph is shown in figure 7.
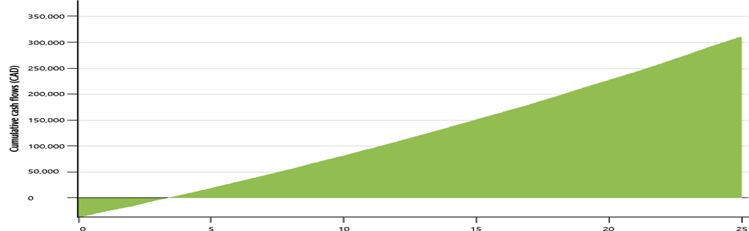
Environmental Analysis
This section aims to understand the impact of extreme weather conditions on the performance of solar PV generating facilities.
Climate change continues to alter typical weather and climate patterns, with the increasing prevalence of extreme weather events, including longer, more intense heat waves and drought conditions. BC strategic climate risk assessment has identified 15 risk events and estimated the likelihood of occurring (both in the present day and in the 2050s) to determine how climate change influences the likelihood of fixed-magnitude events. The results show that wildfire season and heatwave are among the greatest risks which threaten BC by 2050. According to this research, Canada is already experiencing the effects of global climate change: average temperatures are increasing, sea levels are rising, and variable and extreme weather is becoming more frequent
In 2021, British Columbia and Abbotsford, in particular, experienced:
- Heat record of 42°C in June (World Weather, 2021)
- Destructive spread of wildfires in August (Wildfire Season Summary, 2021)
- Unprecedented rain in November led to record-breaking rainfall and catastrophic flooding (Global News, 2021)
Wildfire: Studies show that environmental variables including wildfires, clouds, dust, and other forms of air pollution affect the overall performance of solar PVs. The reason is that wildfire smoke and other particulate matter can substantially inhibit solar photovoltaic (PV) generation production. This section mainly intended to investigate the relationship between effective solar photovoltaic (PV) capacity and wildfire smoke using high aerosol optical density or PM2.5 to quantify the amount of smoke present at a specific point in time. Aerosol optical density determines how much direct sunlight is prevented from reaching the ground by these aerosol particles. Figure 8 shows the effect of air quality (PM2.5 and PM10) on the system’s production.
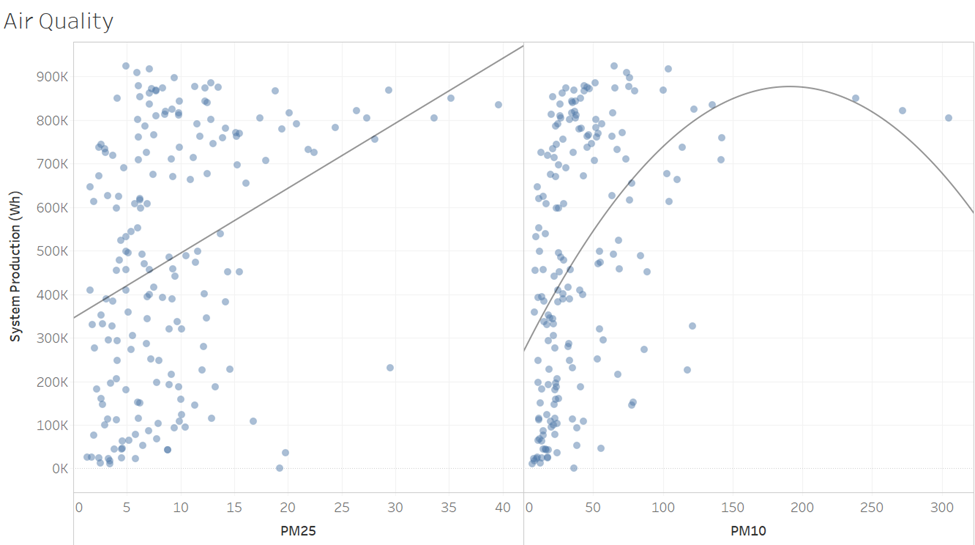
Heat Wave: The production of solar PV generation is directly dependent on meteorological factors such as irradiance, ambient temperature, wind speed, relative humidity, air mass, and their interaction effects. To understand the effect of heat dome on electricity generated by solar PVs in Building G, the climate data and electricity production are obtained from NASA data source and SolarEdge dashboard, respectively. As Figure 9 shows, there is a linear correlation between electricity generated and daily solar radiation (as expected). By increasing temperature, however, the system production tends to experience an exponential function.
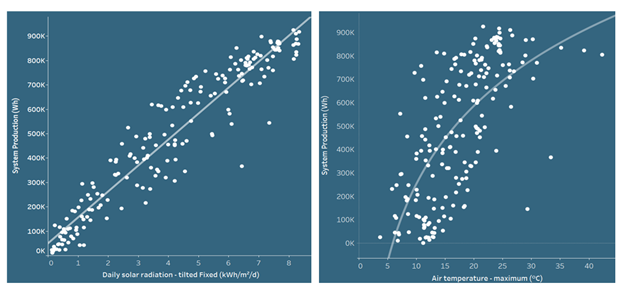
To reduce inaccuracies in estimates of solar PV availability, it is necessary to consider climate change and resulting extreme weather conditions in initial studies. This section primarily intended to investigate the relationship between solar PV capacity and weather conditions through both literature reviews as well as data visualization. Even though extensive research has been carried out to determine the impact of each of these variables on the overall performance (as explained earlier), making a solid conclusion is impossible due to a lack of data. We had one period of heatwave and wildfire while studying the system. It is highly recommended to repeat data analysis with an acceptable amount of data over the following years.
Energy Incentive Programs in British Columbia
The purpose of this section is to provide a review of incentives, rebates, and grants available for institutional buildings in the British Columbia (BC) province. According to the NRCAN website, there are energy incentive programs offered by the Government of Canada, provincial and territorial governments, major Canadian municipalities, and major electric and gas utilities and companies to promote the efficient use or conservation of energy at the end-use level and/or the use of alternative energy in Canada. A list of 24 incentive programs applicable to institutional/commercial buildings (Natural Resources Canada, 2021) have been also included in the main report.
Results and Discussion
Final calculations show that over the next 25 years using renewable energy would lead to about 3.5 GW energy saving which is equivalent to CAD 350K. The total amount of GHG emission reduction is estimated to be 60 tons over the project lifecycle.

Calculations show that even after the energy conservation measure the energy performance metrics would not meet step 2 of the BC energy step code. To meet the minimum criteria, there are three options:
- Combining the solar PV system with other energy ECMs such as other renewable technologies
- Reducing natural gas consumption by replacing equipment with heat pumps and electrical boilers
- Retrofitting the building
For each of these options, a cost-benefit analysis should be done. Then, the final decision would be made based on comparing the cost of each option with the improved energy performance over time.
Furthermore, environmental analysis shows that climate pattern is changing. This means that Solar PV systems may have to work under weather conditions different from what they are testing in laboratories today. So, it is recommended to include different scenarios of weather conditions in feasibility studies as well as contract warranty and guaranty terms for future projects.
As extensive research has been done by Canadian universities on renewable energy sources, investigating the best practices of other campuses in BC such as UVic, UNBC and UBC would help the University of Fraser Valley to take advantage of clean energy projects in the future.
In addition to the above recommendations, this research provides a list of 24 incentive programs applicable to institutional buildings. UFV might be eligible to apply for one or more programs to improve energy efficiency or use of alternative energy in future energy projects in Abbotsford and Chilliwack campuses.
Contact
Parisa Mohammadi
parisa226@gmail.com
+1 (778) 700 6040
https://www.linkedin.com/in/parisa-mohammadi-meng-pmp-6247ab2a/
Acknowledgement
Throughout the writing of this report, I have received a great deal of support and assistance.
I would first like to thank my industry mentor Mr. Mark Goudsblom, the director, campus planning and facilities management at University of the Fraser Valley because of his invaluable expertise in formulating the research questions and methodology.
I would particularly like to thank my professors at University of British Columbia, Dr.Vladan Prodanovic and Dr. Nima Atabaki for their support and insightful feedback that helped me to choose the right direction.
References
2020). Guidance Direct Emissions from Mobile Combustion Source. United States Environmental Protection Agency.
(2021, 10). Retrieved from World Weather: https://world-weather.info/forecast/canada/abbotsford/28-june/
Allouhia, A., El Fouiha, Y., Kousksoub, T., Jamila, A., & Zeraouli, Y. (2015). trends, Energy consumption and efficiency in buildings: current status and future;. Journal of Cleaner Production, 118-130. doi:https://doi.org/10.1016/j.jclepro.2015.05.139.
(2019). BC Energy Step Code. The city of Surrey. doi:https://www.surrey.ca/sites/default/files/corporate-reports/RPT_2018-R179.pdf
Building Codes & Standards. (2021, February 9). Retrieved November 16, 2021, from https://www2.gov.bc.ca/gov/content/industry/construction-industry/building-codes-standards/energy-efficiency
Canada’s action on climate change. (2017). Retrieved 2021, from https://www.canada.ca/en/services/environment/weather/climatechange/climate-action/sustainable-efficient-homes-buildings.html
Canada’s actions to reduce emissions. (2020, September 1). Retrieved September 14, 2021, from https://www.canada.ca/en/services/environment/weather/climatechange/climate-plan/reduce-emissions.html#toc0
Data analysis software and modeling tools. (2021, December 24). Retrieved from https://www.nrcan.gc.ca/maps-tools-and-publications/tools/modelling-tools/retscreen/7465
DONALDSON, D. L., & JAYAWEERA, D. (2021, May 27). Temporal Solar Photovoltaic Generation Capacity Reduction From Wildfire Smoke. IEEE Access, 9. doi:10.1109/ACCESS.2021.3084528
Duncan, S. (2021, November 19). British Columbia at the epicenter of climate change-fuelled weather extremes. Retrieved from EuroNews: https://www.euronews.com/green/2021/11/19/british-columbia-at-the-epicenter-of-climate-change-fuelled-weather-extremes
EIA Today in Energy. (2020, September 19). Retrieved from US Energy Information Administration: https://www.eia.gov/todayinenergy/detail.php?id=45336
Energy Modeling Guidelines. (2018, July 11). Retrieved 12 2, 2021, from City of Vancouver: https://vancouver.ca/files/cov/guidelines-energy-modelling.pdf
Environment and Climate Change Canada. (2021, July 12). Retrieved September 8, 2021, from https://www.canada.ca/en/environment-climate-change/news/2021/07/government-of-canada-confirms-ambitious-new-greenhouse-gas-emissions-reduction-target.html
Global News. (2021). Retrieved from Weather: https://globalnews.ca/news/8438007/bc-year-in-review-weather-extremes/
IEA. (2016). Energy, Climate Change & Environment: 2016 Insights. Paris: IEA.
Li, D., Xu, X., Chen, C.-f., & Menassaa, C. (2019, May). Understanding energy-saving behaviors in the American workplace: A unified theory of motivation, opportunity, and ability. Energy Research & Social Science, 51, 198-209.
McFarlane, B. (2017). Abbotsford Campus Building G Solar Photovoltaic System Proposal. Abbotsford: UFV.
(2017). Metrics Research. BC Housing and the Energy Step Code Council.
Natural Resources Canada. (2021, 11 20). Retrieved from https://oee.nrcan.gc.ca/corporate/statistics/neud/dpa/policy_e/results.cfm?attr=0
Njoku, H. O., & Omeke, O. M. (2020). Potentials and financial viability of solar photovoltaic power generation in Nigeria for greenhouse gas emissions mitigation. Clean Technologies and Environmental Policy, 481–492. doi:https://doi.org/10.1007/s10098-019-01797-8
NRCan, Energy Data and Analysis. (n.d.). Retrieved September 27, 2021, from https://www.nrcan.gc.ca/science-and-data/data-and-analysis/energy-data-and-analysis/energy-facts/energy-and-greenhouse-gas-emissions-ghgs/20063
Owolabi, A. B., Kig, B. E., Roh, J. W., Suh, D., & Huh, J. S. (2020). Measurement and verification analysis on the energy performance of a retrofit residential building after energy efficiency measures using RETScreen. Alexandria Engineering Journal, 4643–4657.
Preliminary Strategic Climate Risk Assessment. (2019, July). Retrieved from BC. Ministry of Environment and Climate: https://www2.gov.bc.ca/assets/gov/environment/climate-change/adaptation/prelim-strat-climate-risk-assessment.pdf
The BC Step Code. (2021). Retrieved 2021, from https://www2.gov.bc.ca/gov/content/industry/construction-industry/building-codes-standards/energy-efficiency
Wah Thong, L., Murugan, S., Kiat Ng, P., & Cha Chee, S. (2016). Analysis of Photovoltaic Panel Temperature Effects on its Efficiency. 2nd International Conference on Electrical Engineering and Electronics Communication System.
Weidaa, S., Kumarb, S., & Madlene, R. (2016). Financial viability of grid-connected solar PV and wind power systems in Germany. 1st Energy Economics Iberian Conference EEIC, 106, pp. 35 – 45. Lisbon.
Wildfire Season Summary. (2021). Retrieved from BC Government: https://www2.gov.bc.ca/gov/content/safety/wildfire-status/about-bcws/wildfire-history/wildfire-season-summary