This is the first in a series of posts on simulating climates in growth chambers. See the end of this post for a full list of entries in this series.
Our lab group investigates genetic variation that is meaningful to local adaptation, with a focus on trees. This is especially important in the context of climate change: long-lived trees may become maladapted over time to the local climate. To what extent are trees locally adapted, and to what extent does plasticity enable them to deal with a variety of circumstances? Valuable information is provided by long-term “provenance trials” in the field and nursery-type “common gardens”, in which a collection of genotypes from various sources is grown in the same environment, or set of environments. The weather in such environments varies from year to year and is not under our control. Over a longer term, the impact of this varying weather is expected to average out and represent the local climate. Yet there will always be some extreme event, perhaps an unusually late spring frost, a summer heat wave, a prolonged drought, or perhaps a very wet rainy season, which affects growth and survival in field trials. And these extreme events have a large effect on ‘adaptation’ in the genetic sense: natural selection for fitness.
WHY ?
Wishing to free ourselves from the vagaries of weather and to test our plant materials in warmer environments than those presently available in the field, we arrive at the controlled climate chamber. There are many restrictions inherent in using growth chambers. They are expensive. Only a limited number of genotypes can be observed over a short period of time. And simulating realistic winters has so far eluded us. This method should be regarded as complementary to field trials, and not a substitute.
HOW ?
How does one go about programming a controlled climate chamber to achieve a set goal? Which temperatures, light intensities and day lengths do we program? From which data set do we derive relevant climate variables, which are those variables, what range do we wish to cover, and is the resulting program actually realistic?
Choosing a baseline data set
We use data from Canadian Climate Normals of 1961-1990, the earliest data set of sufficient coverage and detail.This represents the climate before significant warming occurred, and it is the climate we assume the trees have adapted to through natural selection.
Choosing relevant climate variable(s) and a range you wish to cover
In our first experiments we studied interior lodgepole pine (Pinus contorta ssp. latifolia) populations. For this species, mean annual temperature (MAT) of the seed source is the variable revealing the strongest patterns of correlation with growth (Wang et al. 2006). ClimateWNA (Wang et al. 2012) data were used to plot the map of MAT below.
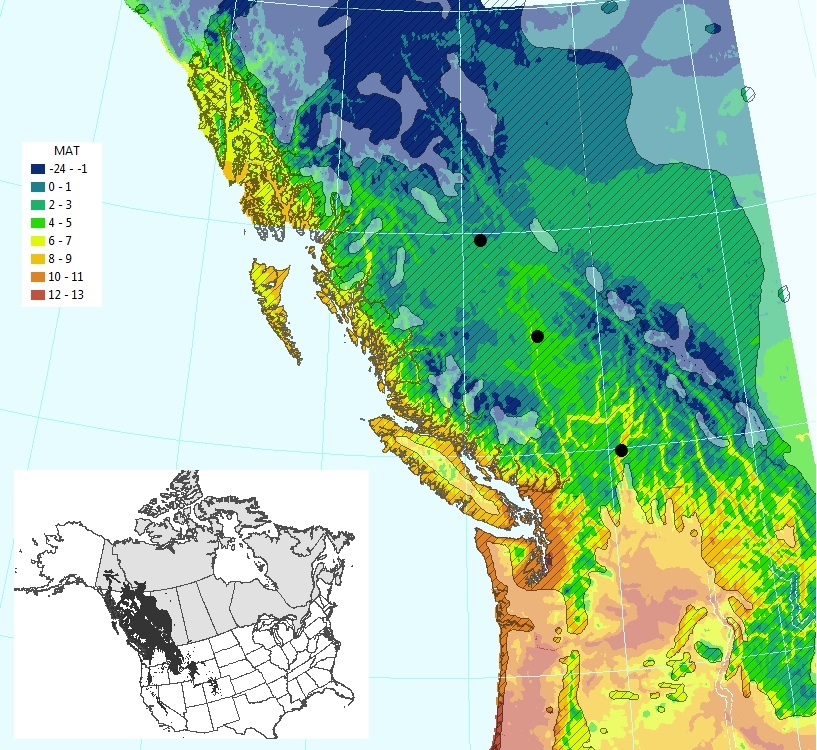
Natural range of Pinus contorta in North America (insert, dark grey) and detail of climate conditions (MAT, °C) in the area of interest (British Columbia, excluding the milky-white overlay where the species does not occur). Three chosen locations with representative climates (black dots).
Our range of interest was interior British Columbia (BC), MAT 1 to 10 °C, to fully capture the growth response functions derived from field data (Wang et al. 2006). The geographic range for ssp. latifolia does not incorporate any areas with MAT 10°C, so this warmer, future climate regime was created by extrapolation. No attempt was made to average the climate for all the points with the same MAT. Instead, three points were chosen on the map, with MAT close to 1, 4 and 7°C, which had weather stations nearby. Such proximity is not really required, since ClimateWNA also provides detail on average daily ranges, but is still useful to inform us about the absolute maximum temperature. Other species may require other approaches, depending on the climate variables that best explain their adaptation. Now that a target climate has been chosen, find out how I developed realistic temperature regimes, moisture regimes, and where you can find suitable photoperiod data. Over the next several days, we’ll be posting on simulating winter conditions, heat waves, stimulating germination and bud set. At the end of the story, you will be familiar with most of the methods we had to use for growing plants for our AdapTree project. If you have tried similar or even stranger things, let me know. I’m curious to find out!
Simulating climates in growth chambers
Note: entries in this list will become available as posts go live between June 1 and June 8
Wang, T., A. Hamann, A. Yanchuk, G. A. O’Neill and S. N. Aitken. 2006. Use of response functions in selecting lodgepole pine populations for future climates. Global Change Biology 12: 2404–2416. Wang, T., A. Hamann, D.L. Spittlehouse and T. Murdock. 2012. ClimateWNA – High-Resolution Spatial Climate Data for Western North America. Journal of Applied Meteorology and Climatology, 51: 16-29.