Go into the UBC chemical storeroom, and you will find a range of chemicals. But what you won’t find at UBC, or anywhere else, is the element unquadseptium. And that’s because unquadseptium, with an atomic number of 147, has yet to be proven to exist and, according to some chemists, will never exist.
Since Dimitri Mendeleev developed the periodic table in 1875, chemists have added 55 elements to its rows and periods. While most of the 118 elements we are familiar with today exist naturally, some exist briefly and only after the collision of high-speed particles. These “synthetic” elements include einsteinium (atomic number 99) through organessan (atomic number 118).
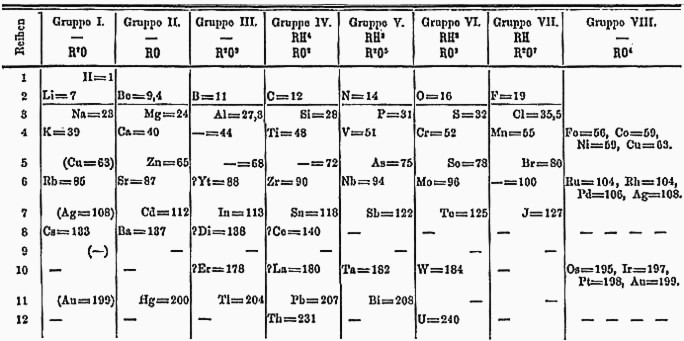
Dimitri Mendeleev’s prototype for the periodic table. Source
Creating new elements is an ongoing area of research. The question that divides chemists is whether a limit exists for nuclear mass and, therefore, the number of elements that may exist. Multiple chemists have used Einstein’s theory of relativity to try and determine the limit to the mass of an atom’s nucleus.
The nucleus of an atom exerts a gravitational and magnetic pull on the orbiting electrons. As the mass of the nucleus grows, so does its pull on the electrons, and as modeled by the Bohr equation, orbiting electrons must travel faster to prevent falling inwards. According to Einstein’s theory of relativity, mass increases exponentially with speed. A result of this relationship is that the speed of light presents a universal speed limit for matter.
This universal limit led Richard Feynman and other chemists to propose element 137 as the limit to the periodic table. Feynman argued that beyond element 137, electrons would have to travel faster than the speed of light to remain in orbit and could not exist according to the laws of physics.
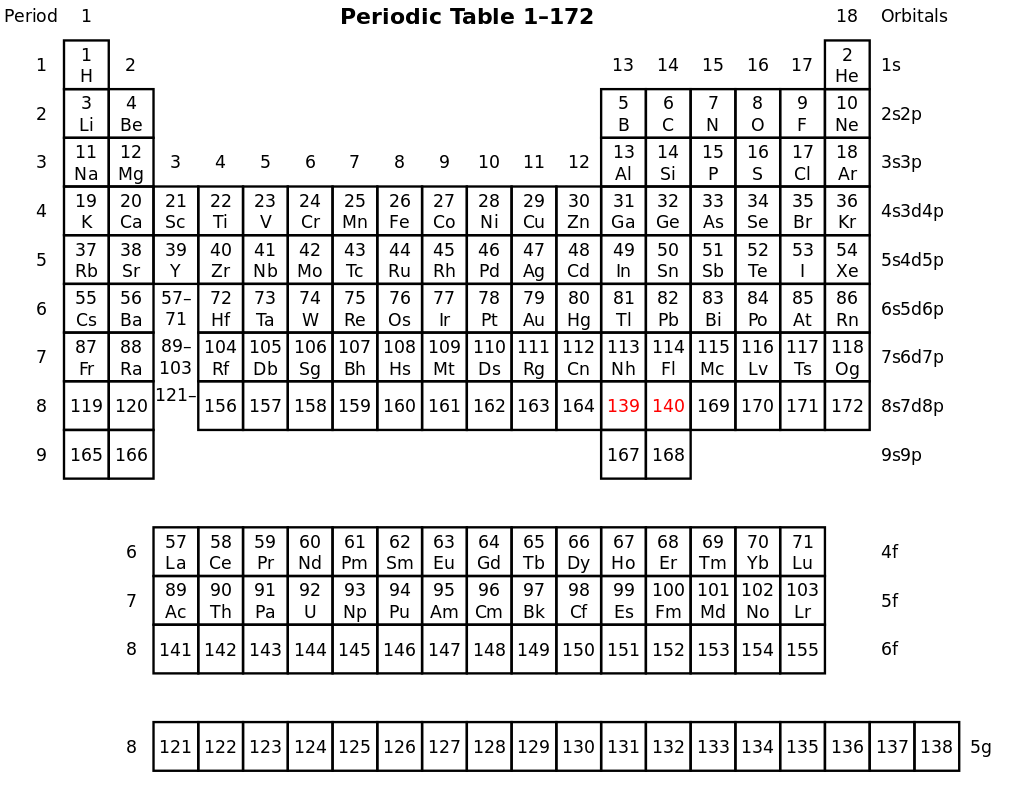
Pekka Pyykkö’s proposed 172-element periodic table. Source
However, many chemists argue that the limit for nuclear mass should be much higher. Notably, Pekka Pyykkö from the University of Helsinki published a paper in 2011 that theorized the existence of elements up to atomic number 172. His paper built upon the work of physicists Berndt Muller and Johann Rafelski. The two physicists used the Dirac equation, which considers effects ignored by the Bohr equation, to find the maximum limit for nuclear mass. According to the Dirac equation, orbiting electrons reach the speed of light when the atomic number equals 173 and not 137.
Pushing the limits of the periodic table further still, some chemists and physicists believe that nuclear mass is unlimited. They propose that new quantum behavior of electrons, unknown to present science, allows the orbit of “superheavy” nuclei. Physicist Walter Greiner believes that after element 172, electrons enter a never-ending continuum of negative energy. Greigner believes that the periodic table “will never end!”
In the coming years, chemists and physicists will discover new elements as we develop stronger particle accelerators and detectors with greater sensitivity. However, it remains unknown what the limit to these discoveries will be; only time will tell whether future chemists will see the likes of unquadseptium in their labs.