Gene therapy is a promising medical development that can save people suffering in perilous diseases. One outstanding case of gene therapy is transplanting transgenic skin cells to replace over 80% (0.85 m2) of a young boy’s outer layer of skin (called the epidermis), effectively treating a severe skin disease called junctional epidermolysis bullosa (JEB) without long-term adverse effects. This accomplishment was published by Professor Michele de Luca and the collaborating medical team from Ruhr-Universität Bochum’s burn unit and the Center for Regenerative Medicine at the University of Modena (Italy), on November 8, 2017, in the journal Nature.
JEB is a disease caused by mutations in any of three genes that encodes a protein called laminin, which anchors the epidermis to the dermis, the inner layer of skin. Failure to do so results in fragile skin with low mechanical resistance and elasticity, manifesting as blisters and wounds in many areas and increased vulnerablility to infections. The patient in the study had extremely severe JEB caused by mutation in one gene and lost an amount of skin equal 80% of his total body surface area, including arms, legs, chest and back.
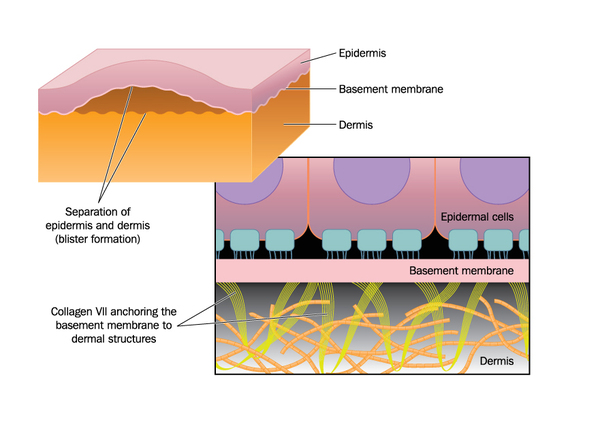
mutation in the laminin protein (not shown) causes detachment of the epidermis (outer skin) from the basemebt membrane and inner skin (dermis) in junctional epidermolysis bullosa. source: https://ghr.nlm.nih.gov/condition/junctional-epidermolysis-bullosa
The treatment started two years ago in 2015, with doctors removing a small area of normal skin to establish skin cultures, which were artificially infected (transduced) with a virus (called a retroviral vector) carrying the normal laminin-encoding gene. After growing the cells to 0.85 m2 , the new skin was sequentially grafted on sites of exposed inner skin, using either plastic or fibrin as the adhesive base. Both graft types were equally effective: the regenerated skin did not blister or damage after pinching; furthermore, after 21 months followup, the grafted skin did not produce antibodies by the body, indicating it was safe and the body recognizes it as belonging to itself. The new skin regenerates monthly by a small number (about 5% of the skin after 8 months) of long-lived stem cells called holoclones, which could regenerate themselves and develop into half-differentiated cells (meroclones) and almost fully differentiated cells (paraclones), which cannot divide further but replace old cells and gradually disappear.
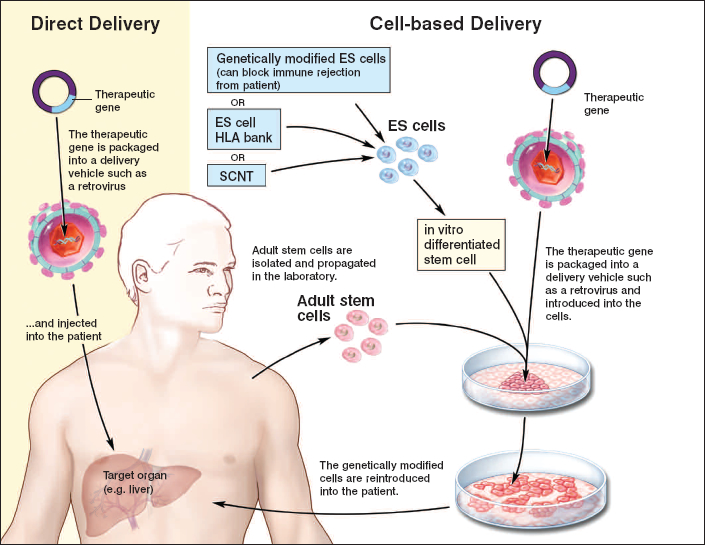
general stem cell therapy scheme. Skin cells from the patient are harvested and a virus introduces the desired gene into the skin culture to create genetically modified cells. Source: https://stemcells.nih.gov/info/Regenerative_Medicine/2006Chapter4.htm
However successful it was, the treatment was actually pretty risky. The retroviral vector could insert, or integrate, the normal laminin gene anywhere in the patient’s genetic blueprint and disrupt other normal genes and cause unregulated tissue growth, resulting in tumours or cancers. Fortunately, genetic sequencing of the transplanted skin revealed that the normal gene was inserted mostly in regions not coding for proteins (introns and intergenic regions) with only 5% inserted in protein-encoding regions, but these genes were not involved in cancer. In addition, the transplant did not cause specific cells to survive better than others and cause tumour formation. However, long term monitoring is still required.
While this technology is currently limited to injuries with an intact dermis, it is less invasive and more effective than surgery as it does not entail infections, and it could be applied to early diagnosis stages to prevent skin diseases as well as restoring large areas of damaged skin. This is a new treatment for epidermolysis bullosa, a condition affecting 500 000 people worldwide, and a major stepping stone to developing stem-cell therapies for many debilitating diseases.
Written by Jenny Zhong