Speculate on how such networked communities could be embedded in the design of authentic learning experiences in a math or science classroom setting or at home. Elaborate with an illustrative example of an activity, taking care to consider the off-line activities as well
The use of virtual field trips and interactive virtual expeditions (IVE) are valuable tools that an educator can use to make science come alive in the classroom. While there is inherent value in students actually going on a field trip the logistics are often daunting. In my school district students arrive at 8:50 am and dismiss at 3: 30 pm. (asking for the bus to arrive early or return late is a logistical nightmare as 95% of our students are bused home and many come from homes with commuting parents or working farms. If we miss the home busses parents need to pick up their child at the school which often means we end up waiting up to 2 hours for parents who are late for the pick up).
We are located in a rural community in the Niagara region of Ontario. Often field trips become social outings rather than educational experiences. Students spend at least two hours on the bus in each direction, which leaves approximately two hours for exploration and lunch. The cost of bussing has become so high the average field trip costs in excess of 40 dollars per child an amount many of our families cannot afford. So, we must weigh the costs and benefits. Often time the costs outweigh the benefits.
Virtual field trips and IVE are life savers for schools like mine. Students enjoy them, learn from them and often continue to explore them on their own time. Niemitz et al (2008) report that “the use of interactive virtual expeditions in classroom learning environments can theoretically be an effective means of engaging learners in understanding science as an inquiry process, infusing current research and relevant science into the classroom, and positively affecting learner attitudes towards science as a process and a career (p. 562).”
The researchers report that studies have shown that virtual field trips can enhance learning (Cox & Su, 2004; Tuthill & Klemm, 2002; Woerner, 1999), achieve the same gains in student achievement as physical field trips (Garner & Gallo, 2005), and provide an effective supplement to physical field trips (Spicer & Stratford, 2001). As such, we can apply many of the best practices of effective virtual field trips (Klemm & Tuthill, 2003; Woerner, 1999) – purposeful trip planning, learner-centered experiences, active student learning, cooperative learning activities, teachers as guides who scaffold learning experiences, differentiated instruction, and multiple opportunities for learner success – to the field of IVE. (Niemitz et al, 2008 p. 566).”
Collaboration amongst students is possible on the virtual reality field trip as much as on a traditional field trip. Often collaboration in both settings provide students with the opportunity to question and test their hypothesis, discuss findings and eliminate misconceptions. According to Driver et al (1994) “Scientific knowledge is socially constructed, validated and communicated (p. 11).” While Lamon, Laferriere & Breuleux, (in press) reported that research shows that knowledge construction is rarely done in isolation but rather by creating and forming a knowledge building community and the goal for learning communities is that a group of students with focused common issues complete tasks better than any single person.
Working collaboratively in math and science requires three important personal characteristics:
INTELLECTUAL COURAGE: we should be ready to revise any one of our beliefs.
INTELLECTUAL HONESTY: we should change a belief when there is a good reason to
change it…
WISE RESTRAINT: we should not change a belief wantonly, without some good reason, without serious examination (Lampert, 1990 pp. 7-8).
Collaboration among students and access to virtual learning environments need to become integral parts of our daily classrooms. After exploring several of the websites this week GLOBE, Exploratorium and virtual field trips I was reminded of a project Trish Roffey and I created last term in ETEC 565A. This project required us to create a google classroom module for the subject and age group of our choice. We chose Engineering for grade three students ( this module could easily be used with almost any grade level). What I was reminded of was that given today’s technology we can create our own virtual reality digital stories and field trips.
Trish used a Ricoh Theta 5 camera that films in 3D to create a virtual tour of the amusement park at the West Edmonton Mall. Her module was based on an end project where students had to design a ride or “car” that would accommodate a special needs classmate. The classmate wanted to enjoy the amusement park as well. All kids could relate to that.
The video can be accessed via https://www.thinglink.com/video/850811682614673410
It is best watched using google cardboard or on a tablet (many laptops will not display it properly).
I created a video that took students to the plains in Africa where a young student had made his own wind turbine from found materials. This turbine solved many issues for his family including refrigeration and crop irrigation. In the video, studetns saw the geography, weather patterns and crop growth for the area. They like the boy in the story had to create a device from found materials that would solve a social justice issue in any area of the world.
All that being said what Trish and I found to be the best “gotcha” with the students is that they were not just expected to learn new information but they had to work together to solve a problem. This made the learning real and valuable and students saw the connections to real life.
Here are some screen shots of our Google classroom:
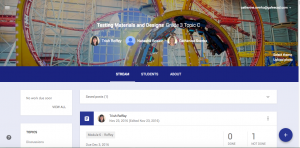
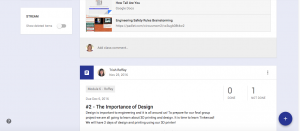
If you would like to look at it more in-depth or look at the entire module contact me and I will provide a user name and password.
Catherine
References:
Driver, R., Asoko, H., Leach, J., Scott, P., & Mortimer, E. (1994). Constructing scientific knowledge in the classroom. Educational researcher, 23(7), 5-12.
Falk, J. & Storksdieck, M. (2010). Science learning in a leisure setting. Journal of Research in Science Teaching, 47(2), 194-212.
Lamon, M., & Laferrière, T., & Breuleux, A. (in press). Networked communities. In P. Resta, Ed., Teacher development in an e-learning age: A policy and planning guide, UNESCO.
Lampert, M. (1990). When the problem is not the question and the solution is not the answer: Mathematical knowing and teaching. American educational research journal, 27(1), 29-63.
Niemitz, M., Slough, S., Peart, L., Klaus, A., Leckie, R. M., & St John, K. (2008). Interactive virtual expeditions as a learning tool: The School of Rock Expedition case study. Journal of Educational Multimedia and Hypermedia, 17(4), 561-580.